5 Meet the Living Primates
Beth Shook; Ph.D.; Lara Braff; Katie Nelson; Kelsie Aguilera; and M.A.
Stephanie Etting, Ph.D., Sacramento City College
This chapter is a revision from “Chapter 5: Meet the Living Primates” by Stephanie Etting. In Explorations: An Open Invitation to Biological Anthropology, first edition, edited by Beth Shook, Katie Nelson, Kelsie Aguilera, and Lara Braff, which is licensed under CC BY-NC 4.0.
Learning Objectives
- Describe how studying nonhuman primates is important in anthropology.
- Compare two ways of categorizing taxa: grades and clades.
- Define different types of traits used to evaluate primate taxa.
- Identify key ways that primates differ from other mammals.
- Distinguish between the major primate taxa using their key characteristics.
- Describe your place in nature by learning your taxonomic classification.
You may be wondering why a field dedicated to the study of humans includes discussions of nonhuman animals. Our primary goal in biological anthropology is to understand how humans are similar to and different from the rest of the natural world, why we have the traits we have, and how we got to be the way we are. But to fully grasp our place in nature, we must look to our closest living relatives, the nonhuman primates. In this chapter, we focus on the organization and diversity within the Order Primates.
Studying Primates in Biological Anthropology
Primates are one of at least twenty Orders belonging to the Class Mammalia, and probably one of the oldest. One genetic estimate puts the origin of primates at approximately 91 million years ago (mya), predating the extinction of the dinosaurs (Bininda-Emonds et al. 2007). Today, the Order Primates is a diverse group of animals that includes lemurs and lorises, tarsiers, monkeys, apes, and humans, all of which are united in sharing a suite of anatomical, behavioral, and life history characteristics. While nonhuman primates are fascinating animals in their own right, their close relationship to humans makes them ideal for studying humans via homology, looking at traits that are shared between taxa because they inherited the trait from a common ancestor. For example, humans (genus Homo) and chimpanzees (genus Pan) both share the trait of male cooperation in hunting. This trait—along with many others that chimpanzees and humans share—is likely homologous, meaning it was probably passed down from the last common ancestor of Homo and Pan, which lived about 6–8 million years ago.
Nonhuman primates also make excellent comparators for learning about humans via analogy. Many nonhuman primates live in environments similar to those in which our ancestors lived and therefore exhibit traits similar to what we see in humans. For example, baboons and humans both have long legs. In humans, this is because about 1.7 million years ago, our ancestors moved into savanna habitats where longer legs helped them move more efficiently over long distances. Baboons, who also live in savanna habitats, independently evolved longer arms and legs for the same reason—to be able to cover more ground, more efficiently. This means that having long legs is an analogous trait in baboons and humans: —that is, this adaptation evolved independently in the two species but for the same purpose. Using homology and analogy, our closest living relatives provide the critical context in which to understand human biology, morphology, and behavior. It is only by studying how humans compare with our primate relatives that we can fully comprehend our place in nature.
Ways of Organizing Taxa
You learned in Chapter 2 about Linnaeus and the hierarchical nature of taxonomic classification. Our goal in classifying taxa is to create categories that reflect clade relationships. A clade is a grouping of organisms based on relatedness that reflects a branch of the evolutionary tree. Clade relationships are determined using traits shared by groups of taxa as well as genetic similarities. An example of a clade would be a grouping that includes humans, chimpanzees, bonobos, and gorillas (Figure 5.1). These taxa are in what is referred to as the African clade of hominoids (a taxonomic group you will learn about later in this chapter). The African clade grouping reflects how humans, chimpanzees, bonobos, and gorillas all share a more recent ancestor with each other than any of them do with other species—that is, we are on the same branch of the evolutionary tree. We know members of the African clade are most closely related based on shared morphological traits as well as genetic similarities. Excluded from this grouping is the orangutan, which is considered a member of the Asian clade of hominoids.
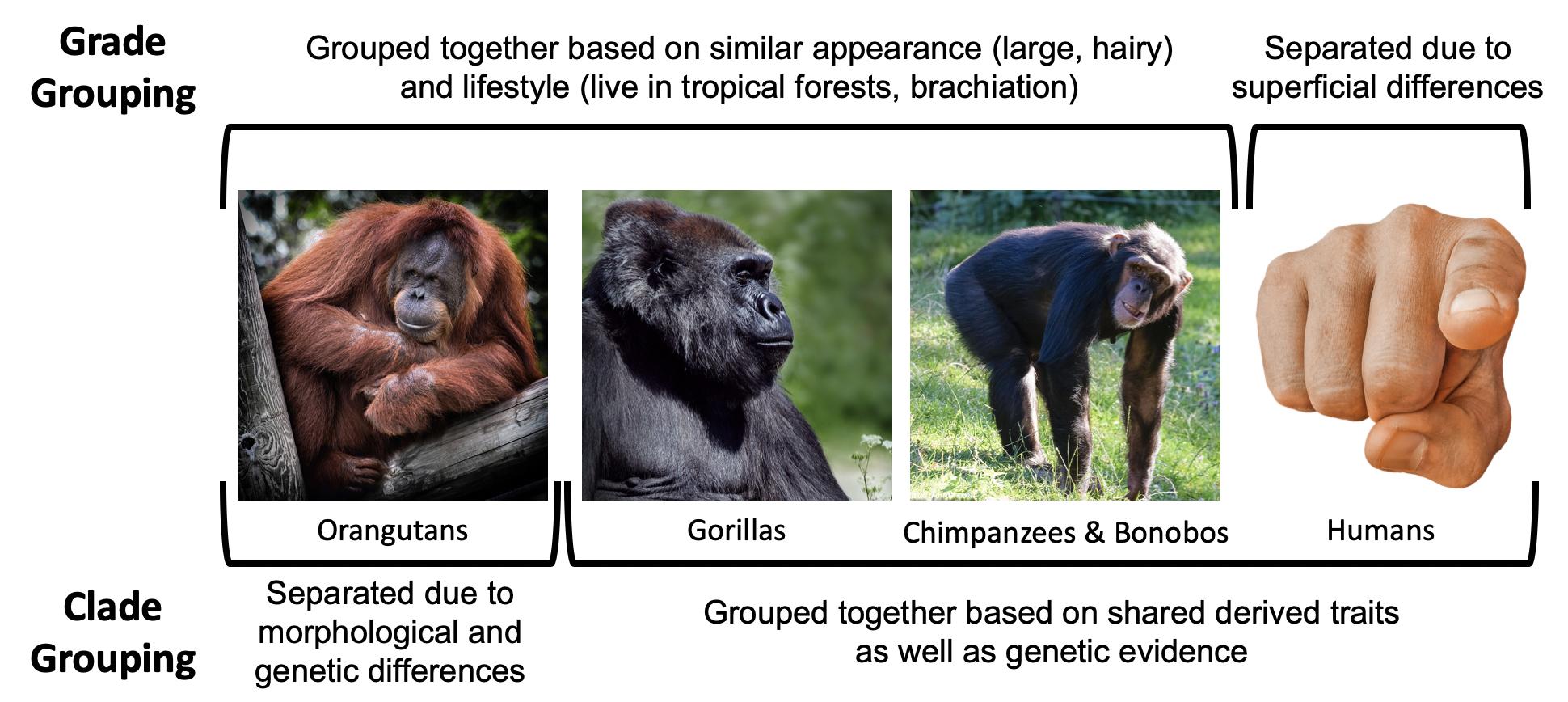
In contrast, grades are groupings that reflect levels of adaptation or overall similarity and not necessarily evolutionary relationships. An example of a grade would be placing orangutans, gorillas, bonobos, and chimpanzees into a group, and excluding humans. Grouping in this way is based on the superficial similarities of the apes in being large-bodied, having lots of body hair, living in tropical forests, climbing and sleeping in trees, and so on. According to these criteria, humans seem to be unusual in that we differ in our morphology, behavior, and ecology. Separating humans from the large-bodied apes is the system that was used historically. We now know that grouping orangutans, gorillas, bonobos, and chimpanzees and excluding humans does not accurately reflect our true evolutionary relationships. Since our goal in taxonomic classification is to organize animals to reflect their evolutionary relationships, we prefer to use clade classifications.
Types of Traits
When evaluating relationships between taxa, we use key traits that allow us to determine which species are most closely related to one another. Traits can be either ancestral or derived. Ancestral traits are those that a taxon has because it has inherited the trait from a distant ancestor. For example, all primates have body hair because we are mammals and all mammals share an ancestor hundreds of millions of years ago that had body hair. This trait has been passed down to all mammals from a shared ancestor, so all mammals alive today have body hair. Derived traits are those that have been more recently altered. This type of trait is most useful when we are trying to distinguish one group from another because derived traits tell us which taxa are more closely related to each other. For example, humans walk on two legs.The many adaptations that humans possess that allow us to move in this way evolved after humans split from the Genus Pan. This means that when we find fossil taxa that share derived traits for walking on two legs, we can conclude that they are likely more closely related to humans than to chimpanzees and bonobos.
There are a couple of other important points about ancestral and derived traits that will become apparent as we discuss primate diversity. First, the terms ancestral and derived are relative terms, meaning that a trait can be either one depending on the taxa being compared. For example, in the previous paragraph, body hair was used as an example for an ancestral trait among primates. All mammals have body hair because we share a distant ancestor who had this trait. The presence of body hair therefore doesn’t allow you to distinguish whether monkeys are more closely related to apes or lemurs because they all share this trait. However, if we are comparing mammals to birds and fish, then body hair becomes a derived trait of mammals. It evolved after mammals diverged from birds and fish, and it tells us that all mammals are more closely related to each other than they are to birds or fish.The second important point is that very often when one lineage splits into two, one taxon will stay more similar to the last common ancestor in retaining more ancestral traits, whereas the other lineage will usually become more different from the last common ancestor by developing more derived traits. This will become very apparent when we discuss the two suborders of primates, Strepsirrhini and Haplorrhini. When these two lineages diverged, strepsirrhines retained more ancestral traits (those present in the earliest primates) and haplorrhines developed more derived traits (became more different from ancestral primates).
There are two other types of traits that will be relevant to our discussions here: generalized and specialized traits. Generalized traits are those characteristics that are useful for a wide range of things. Having opposable thumbs that go in a different direction than the rest of your fingers is a very useful, generalized trait. You can hold a pen, grab a branch, peel a banana, or text your friends all thanks to your opposable thumbs! Specialized traits are those that have been modified for a specific purpose. These traits may not have a wide range of uses, but they will be very efficient at their job. Hooves in horses are a good example of a specialized trait: they allow horses to run quickly on the ground on all fours. You can think of generalized traits as a Swiss Army knife, useful for a wide range of tasks but not particularly good at any one of them. That is, if you’re in a bind, then a Swiss Army knife can be very useful to cut a rope or fix a loose screw, but if you were going to build furniture or fix a kitchen sink, then you’d want specialized tools for the job. As we will see, most primate traits tend to be generalized.
What Makes Something a Primate?
The Order Primates is distinguished from other groups of mammals in having a suite of characteristics. This means that there is no individual trait that you can use to instantly identify an animal as a primate; instead, you have to look for animals that possess a collection of traits. What this also means is that each individual trait we discuss may be found in nonprimates, but if you see an animal that has most or all of these traits, there is a good chance it is a primate.
Primates are most distinguishable from other organisms in traits related to our vision. Our Order relies on vision as a primary sense, which is reflected in many areas of our anatomy and behavior. All primates have eyes that face forward with convergent (overlapping) visual fields. So if you cover one eye with your hand, you can still see most of the room with your other one. This also means that we cannot see on the sides or behind us as well as some other animals can. In order to protect the sides of the eyes from the muscles we use for chewing, all primates have at least a postorbital bar, a bony ring around the outside of the eye (Figure 5.2). Primate taxa with more convergent eyes need extra protection, so animals with greater orbital convergence will have a postorbital plate or postorbital closure in addition to the bar (Figure 5.2).The postorbital bar is a derived trait of primates, appearing in our earliest ancestors.
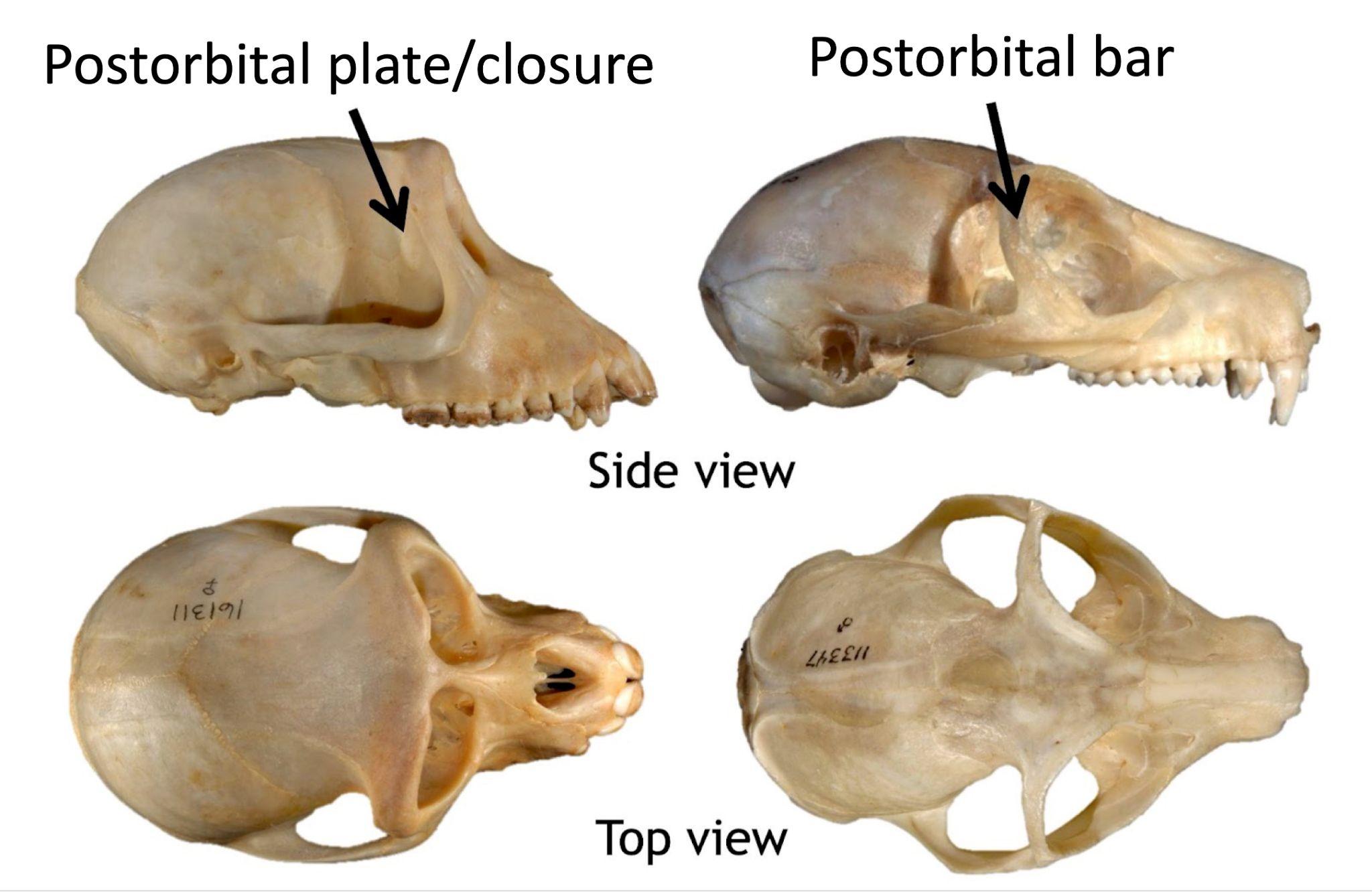
Another distinctive trait of our Order is that many primates have trichromatic color vision, the ability to distinguish reds and yellows in addition to blues and greens. Birds, fish, and reptiles are tetrachromatic (they can see reds, yellows, blues, greens, and even ultraviolet), but most mammals, including some primates, are only dichromatic (they see only in blues and greens). It is thought that the nocturnal ancestors of mammals benefited from seeing better at night rather than in color, and so dichromacy is the ancestral condition for mammals. Trichromatic primates are known to use their color vision for all sorts of purposes: finding young leaves and ripe fruits, identifying other species, and evaluating signals of health and fertility.
The primate visual system uses a lot of energy, so primates have compensated by cutting back on other sensory systems, particularly our sense of smell. Compared to other mammals, primates have reduced snouts, another derived trait that appears even in the earliest primate ancestors. There is variation across primate taxa in how much snouts are reduced. Those with a better sense of smell usually have poorer vision than those with a relatively dull sense of smell. The reason for this is that all organisms have a limited amount of energy to spend on running our bodies, so we make evolutionary trade-offs, as energy spent on one trait cuts back on energy spent on another. So primates with better vision are spending more energy on vision and thus have a poorer smell (and shorter snout), and those who spend less energy on vision will have a better sense of smell (and a longer snout).
Primates also differ from other mammals in the size and complexity of our brains. On average, primates have brains that are twice as big for their body size when compared to other mammals. Not unexpectedly, the visual centers of the brain are larger in primates and the wiring is different from that in other animals, reflecting our reliance on this sense. The neocortex, which is used for higher functions like consciousness and language in humans, as well as sensory perception and spatial awareness, is also larger in primates relative to other animals. In nonprimates this part of the brain is often smooth, but in primates it is made up of many folds, which increase the surface area. It has been proposed that the more complex neocortex of primates is related to diet, with fruit-eating primates having larger relative brain sizes than leaf-eating primates, due to the more challenging cognitive demands required to find and process fruits (Clutton-Brock and Harvey 1980). An alternative hypothesis argues that larger brain size is necessary for navigating the complexities of primate social life, with larger brains occurring in species who live in bigger, more complex groups relative to those living in pairs or solitarily (Dunbar 1998). There seems to be support for both hypotheses, as large brains are a benefit under both sets of selective pressures.
Animals with large brains usually have extended life history patterns, and primates are no exception. Life history refers to the pace at which an organism grows, reproduces, and ages. Some animals grow very quickly and reproduce many offspring in a short time frame but do not live very long. Other animals grow slowly, reproduce few offspring, reproduce infrequently, and live a long time. Primates are all in the “slow lane” of life history patterns. Compared to animals of similar body size, primates grow and develop more slowly, have fewer offspring per pregnancy, reproduce less often, and live longer. Primates also invest heavily in each offspring. With a few exceptions, most primates only have one offspring at a time. A group of small-bodied monkeys in South America regularly give birth to twins, and some lemurs can give birth to multiple offspring at a time, but these primates are the exception rather than the rule. Primates also reproduce relatively infrequently. The fastest-reproducing primates will produce offspring about every six months, while the slowest, the orangutan, reproduces only once every seven to nine years. This very slow reproductive rate makes the orangutan the slowest-reproducing animal on the planet! Primates are also characterized by having long lifespans. The group that includes humans and large-bodied apes has the most extended life history patterns among all primates, with some large-bodied apes estimated to live up to 58 years in the wild (Robson et al. 2006).
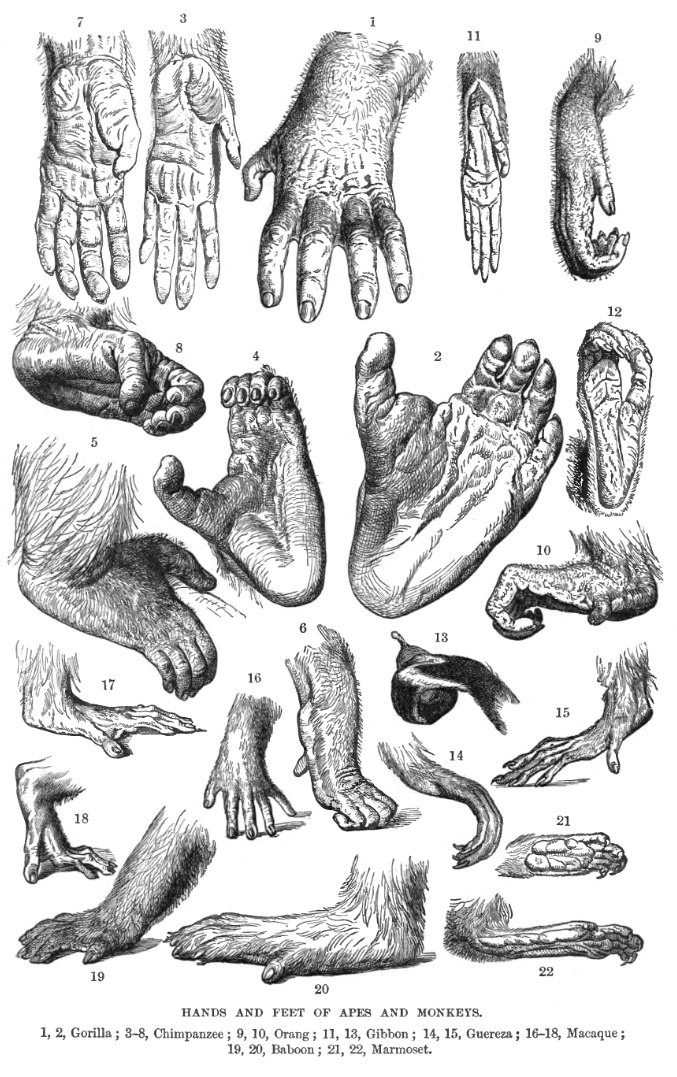
Primates also differ from other animals in our hands and feet. The Order Primates is a largely arboreal taxonomic group, meaning that most primates spend a significant amount of their time in trees. As a result, the hands and feet of primates have evolved to move in a three-dimensional environment. Primates have the generalized trait of pentadactyly— possessing five digits (fingers and toes) on each limb. Many nonprimates, like dogs and horses, have fewer digits because they are specialized for high-speed, terrestrial (on the ground) running. Pentadactyly is also an ancestral trait, one that dates back to the earliest four-footed animals. Primates today have opposable thumbs and, with the exception of humans, opposable big toes (Figure 5.3). Opposable thumbs and toes are a derived trait that appeared in the earliest primate fossils about 55 million years ago. Having thumbs and big toes that go in a different direction from the rest of the fingers and toes allow primates to be excellent climbers in trees as well as to manipulate objects. Our ability to manipulate objects is further enhanced by the flattened nails on the backs of our fingers and toes that we possess in the place of the claws and hooves that many other mammals have. On the other side of our digits, we have sensitive tactile pads that allow us to have a fine sense of touch. Primates use this fine sense of touch for handling food and, in many species, grooming themselves and others. In primates, grooming is an important social currency, through which individuals forge and maintain social bonds.
Primate suite of traits |
Convergent eyes Postorbital bar Many have trichromatic color vision Short snouts Opposable thumbs and big toes Pentadactyly Flattened nails Tactile pads Highly arboreal Large brains Extended life histories Live in the tropics |
Lastly, primates are very social animals. All primates, even those that search for food alone, establish strong social networks within species. Unlike many animals, primates do not migrate: they stay in a relatively stable area for their whole life, often interacting with the same individuals for their long lives. The long-term relationships that primates form with others of their species lead to complex and fascinating social behaviors (see Chapter 6). Finally, nonhuman primates show a clear preference for tropical regions of the world. Most primates are found between the Tropic of Cancer and the Tropic of Capricorn, with only a few taxa living outside these regions. Figure 5.4 shows a summary of primate traits.
Key Traits Used to Distinguish Between Primate Taxa
When placing primate species into specific taxonomic groups, we focus on dental characteristics, behavioral adaptations, and locomotor adaptations. Differences in these characteristics across groups reflect constraints of evolutionary history as well as variation in adaptations.
Dental Characteristics
Teeth may not seem like the most exciting topic with which to start, but we can learn a tremendous amount about an organism from its teeth. First, teeth are vital to survival. Wild animals do not have the benefit of knives and forks; they rely on their teeth to process their food. Because of this, teeth of any species have evolved to reflect what that organism eats and therefore have a lot to tell us about their diet. Second, variation in tooth size, shape, and number reveals an organism’s evolutionary history. Some taxa have more teeth than others or different forms of teeth. Furthermore, differences in teeth between males and females can tell us about competition over mates (see Chapter 6). Lastly, teeth are overly represented in the fossil record. Enamel is hard, and there is little meat on jaws so carnivores and scavengers often leave them behind. Sometimes, the only remains we have from an extinct taxon is its teeth!
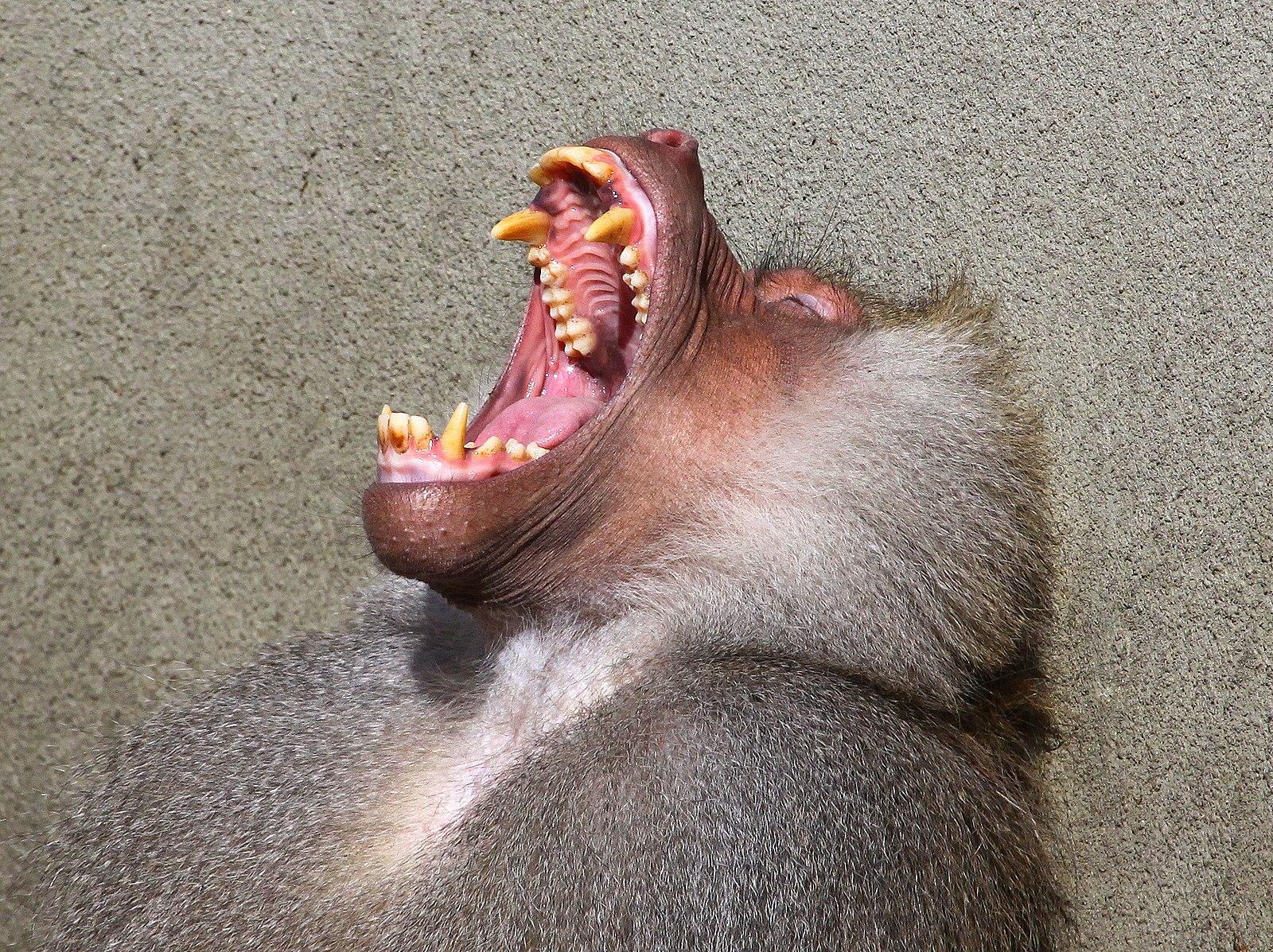
Like other mammals, primates are heterodont: they have multiple types of teeth that are used for different purposes. We have incisors for slicing; premolars and molars for grinding up our food; and canines, which most primates (not humans) use as weapons against predators and each other. The sizes of canines vary across species and can often be sexually dimorphic, with males tending to have larger canines than females. Some nonhuman primates hone, or sharpen, their canines by gnashing the teeth together to sharpen the sides. The upper canine sharpens on the first lower premolar and the lower canine sharpens on the front of the upper canine. As canines get larger, they require a space to fit in order for the jaws to close. This space between the teeth is called a diastema (Figure 5.5).
We use a dental formula to specify how many incisors, canines, premolars, and molars are in each quadrant of the mouth (half of the top or bottom). For example, Figure 5.6 shows half of the lower teeth of a human. You can see that in half of the mandible, there are two incisors, one canine, two premolars, and three molars. This dental formula is written as 2:1:2:3. (The first number represents the number of incisors, followed by the number of canines, premolars, and molars).
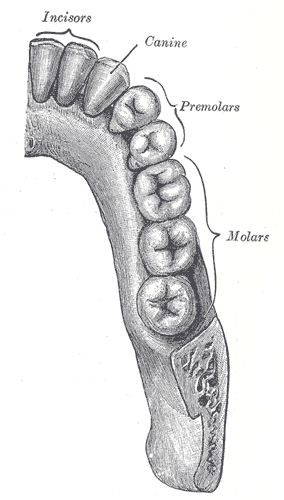
To determine the dental formula, you need to be able to identify the different types of teeth. You can recognize incisors because they often look like spatulas with a flat, blade-like surface. Premolars and molars can be differentiated by the number of cusps that they have. Cusps are the bumps that you can feel with your tongue on the surface of your back teeth. Premolars are smaller than molars and, in primates, often have one or two cusps on them. Molars are bigger, providing a larger chewing surface, and have more cusps. Depending on the species and whether you’re looking at upper or lower teeth, primate molars can have between three and five cusps. Molar cusps can also vary between taxa in how they are arranged; you will learn more about this later in this chapter. Canines are often easy to distinguish because, in most taxa, they are much longer and more conical than the other teeth.
Teeth also directly reflect an organism’s diet. Primates are known to eat a wide range of plant parts, insects, gums, and, rarely, meat. While all primates eat a variety of foods, what differs among primates are the proportions of each of these food items in the diet. That is, two primates living in the same forest may be eating the same foods but in vastly different proportions, and so we would categorize them as different dietary types. The most common dietary types among primates are those whose diets consist primarily of fruit (frugivores), those who eat mostly insects (insectivores), and those who eat primarily leaves (folivores). A few primate taxa are gummivores, specializing in eating gums and saps, but we will only focus on the adaptations found in the three primary dietary groups.
Frugivores
Plants want animals to eat their fruits because, in doing so, animals eat the seeds of the fruit and then disperse them far away from the parent plant. Therefore, plants often “advertise” fruits by making them colorful and easy to spot, full of easy-to-digest sugars that make them taste good and, often, easy to chew and digest (not being too fibrous or tough). For these reasons, frugivores often do not need a lot of specialized traits to consume a diet rich in fruits (Figure 5.7). Their molars usually have a broad chewing surface with low, rounded cusps (referred to as bunodont molars). Frugivores have large incisors for slicing through the outer coatings on fruit, and they tend to have stomachs, colons, and small intestines that are intermediate in terms of size and complexity between insectivores and folivores (Chivers and Hladik 1980). They are also usually of intermediate body size between the other two dietary types. Because fruit does not contain protein, frugivores must supplement their diet with protein from insects, leaves, and/or seeds. Frugivores who get protein by eating seeds evolved to have thicker enamel on their teeth to protect them from excessive wear.
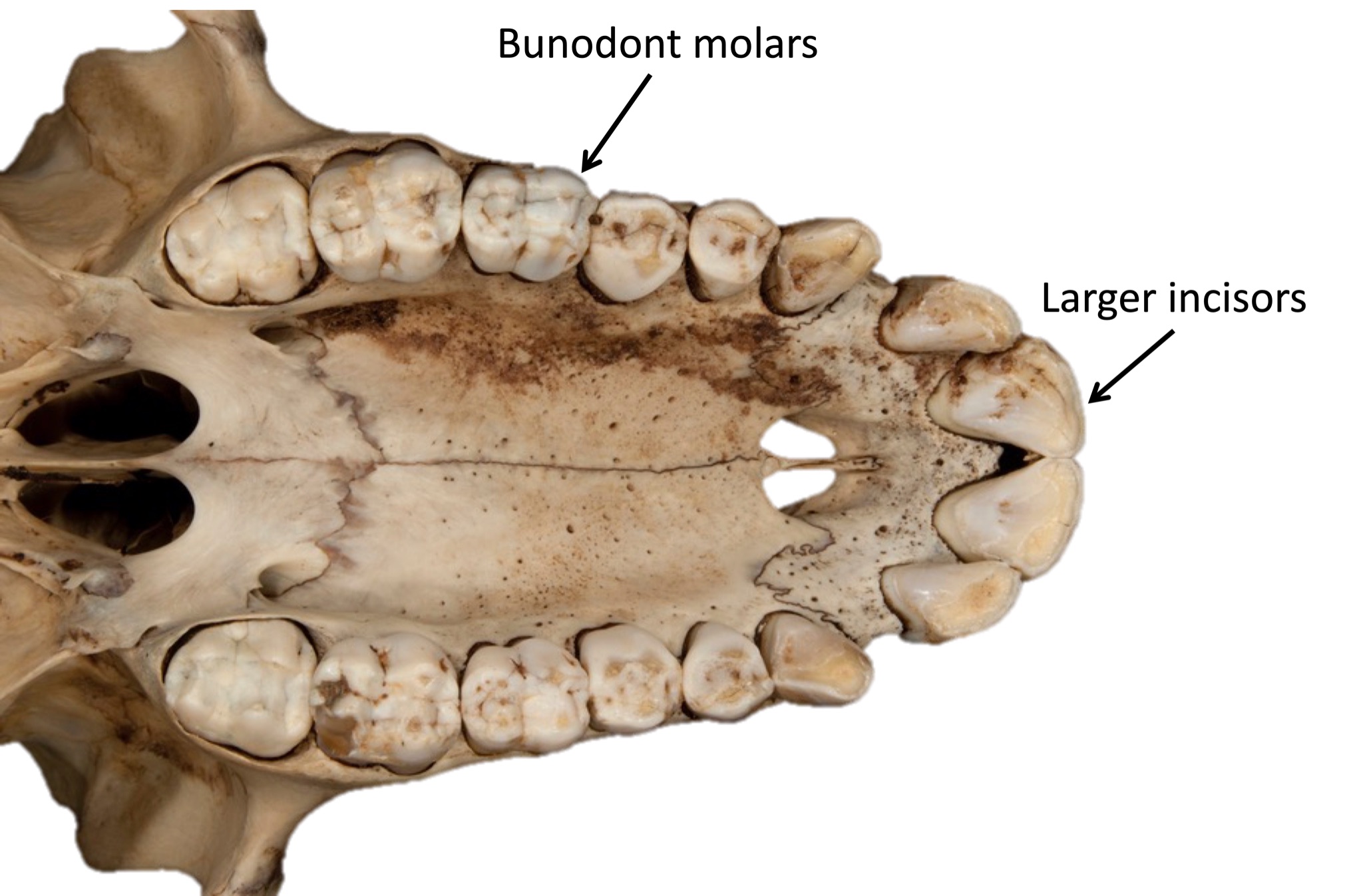
Insectivores
While insects can be difficult to find and catch, they are easy to chew and digest. As a result, insectivorous primates usually have small molars with pointed cusps to puncture the exoskeleton of the insects (Figure 5.8), and they have simple stomachs and colons with a long small intestine to process the insects. Nutritionally, insects provide a lot of protein and fat but are not plentiful enough in the environment to support large-bodied animals, so insectivores are usually the smallest of the primates.
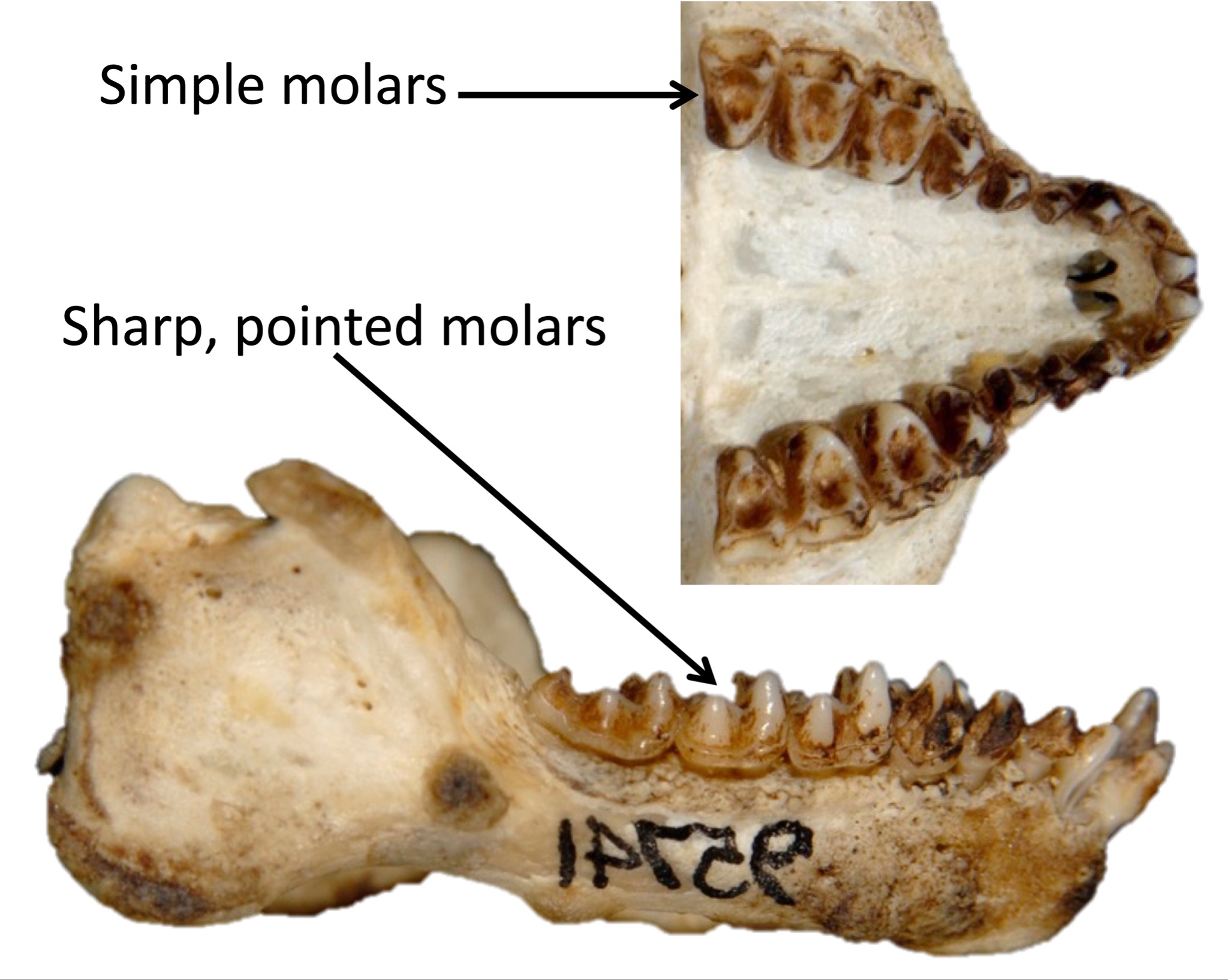
Folivores
Plants rely on leaves to get energy from the sun, so plants do not want animals to eat their leaves (unlike their fruit). As a result, plants evolved to try to discourage animals from eating their leaves. Leaves often carry toxins, taste bitter, are very fibrous and difficult to chew, and are made of large cellulose molecules that are difficult to break down into usable sugars. Thus, animals who eat leaves need a lot of specialized traits (Figure 5.9). Folivorous primates have broad molars with high, sharp cusps connected by shearing crests. These molar traits allow folivores to physically break down fibrous leaves when chewing. Folivores then chemically break down cellulose molecules into usable energy. To do this, some folivores have complex stomachs with multiple compartments, while others have large, long intestines and special gut bacteria that can break up cellulose. Folivores are usually the largest bodied of all primates, and they tend to spend a large portion of their day digesting their food, so they are less active than frugivores or insectivores.
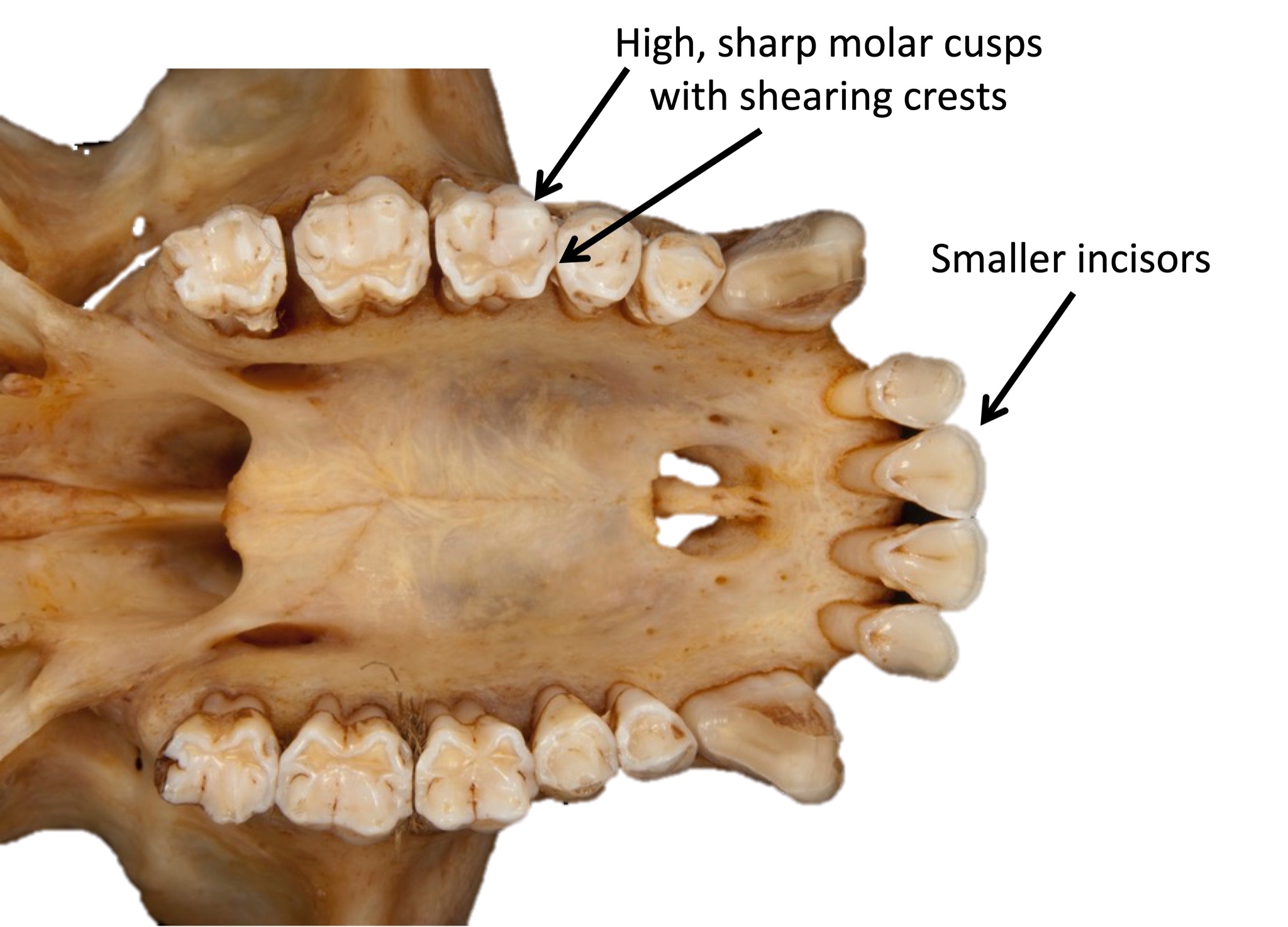
Behavioral Adaptations
Since Chapter 6 is dedicated to primate behavior, we will only briefly discuss variations in activity patterns, social grouping, and habitat use. Primate groups differ in activity patterns: whether they are active during the day (diurnal), at night (nocturnal), or through the 24-hour period (cathemeral). Primate taxa vary in social groupings: some are primarily solitary, others live in pairs, and still others live in groups of varying sizes and compositions. Lastly, some taxa are primarily arboreal while others are more terrestrial.
Locomotor Adaptations
Finally, primate groups vary in their adaptations for different forms of locomotion, or how they move around. Living primates are known to move by vertical clinging and leaping, quadrupedalism, brachiation, and bipedalism.
Vertical clinging and leaping is when an animal grasps a vertical branch with its body upright, pushes off with long hind legs, and then lands on another vertical support branch (Figure 5.10a). Animals who move in this way usually have longer legs than arms, long fingers and toes, and smaller bodies. Vertical clinger leapers also tend to have elongated ankle bones, which serve as a lever to help them push off with their legs and leap to another branch (Figure 5.10b).
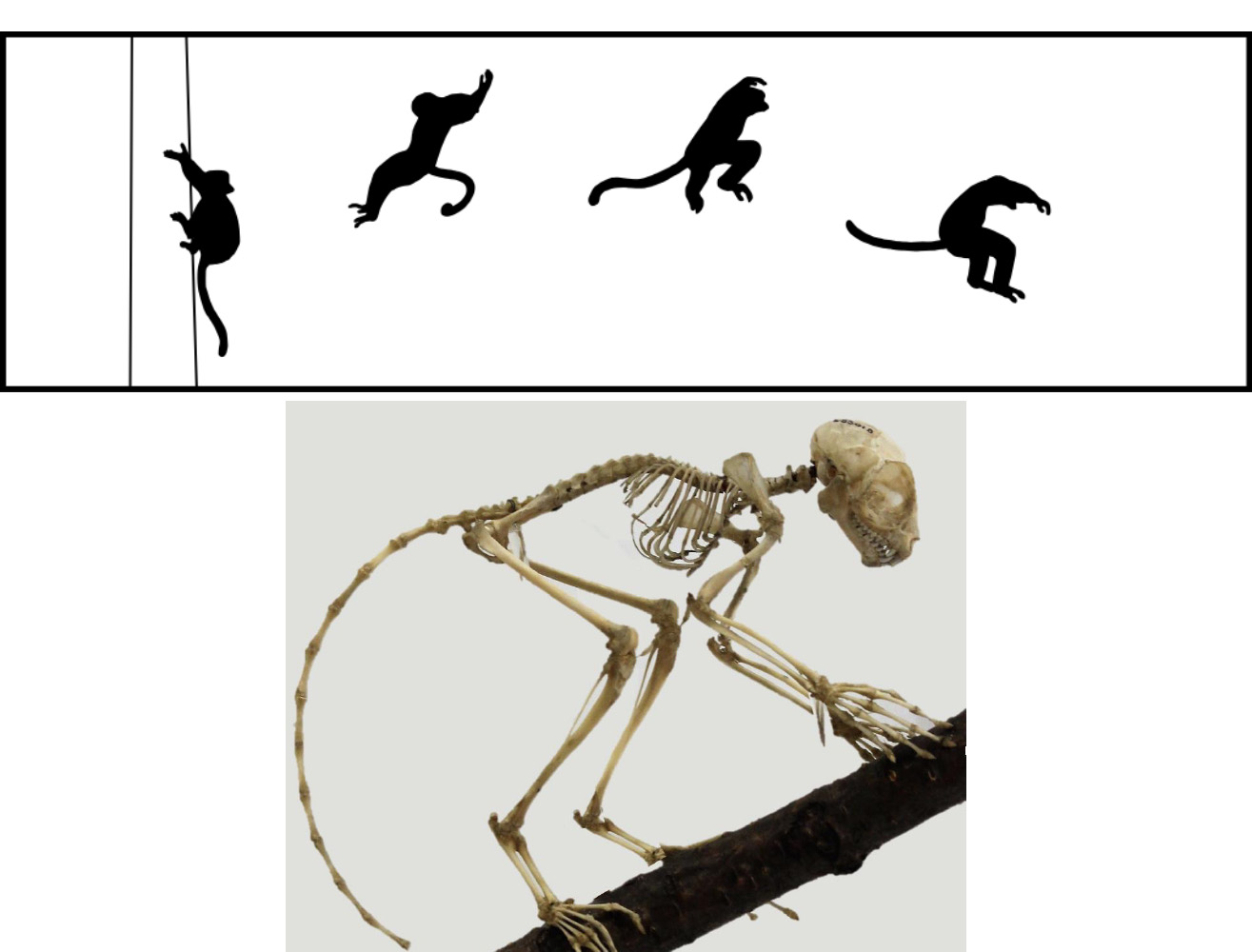
Quadrupedalism, walking on all fours, is the most common form of locomotion among primates. Quadrupedal animals usually have legs and arms that are about the same length and a tail for balance. Arboreal quadrupeds (Figure 5.11a) usually have shorter arms and legs and longer tails, while terrestrial quadrupeds (Figure 5.11b) have longer arms and legs and, often, shorter tails. These differences relate to the lower center of gravity needed by arboreal quadrupeds for balance in trees and the longer tail required for better balance when moving along the tops of branches. Terrestrial quadrupeds have longer limbs to help them cover more distance more efficiently.
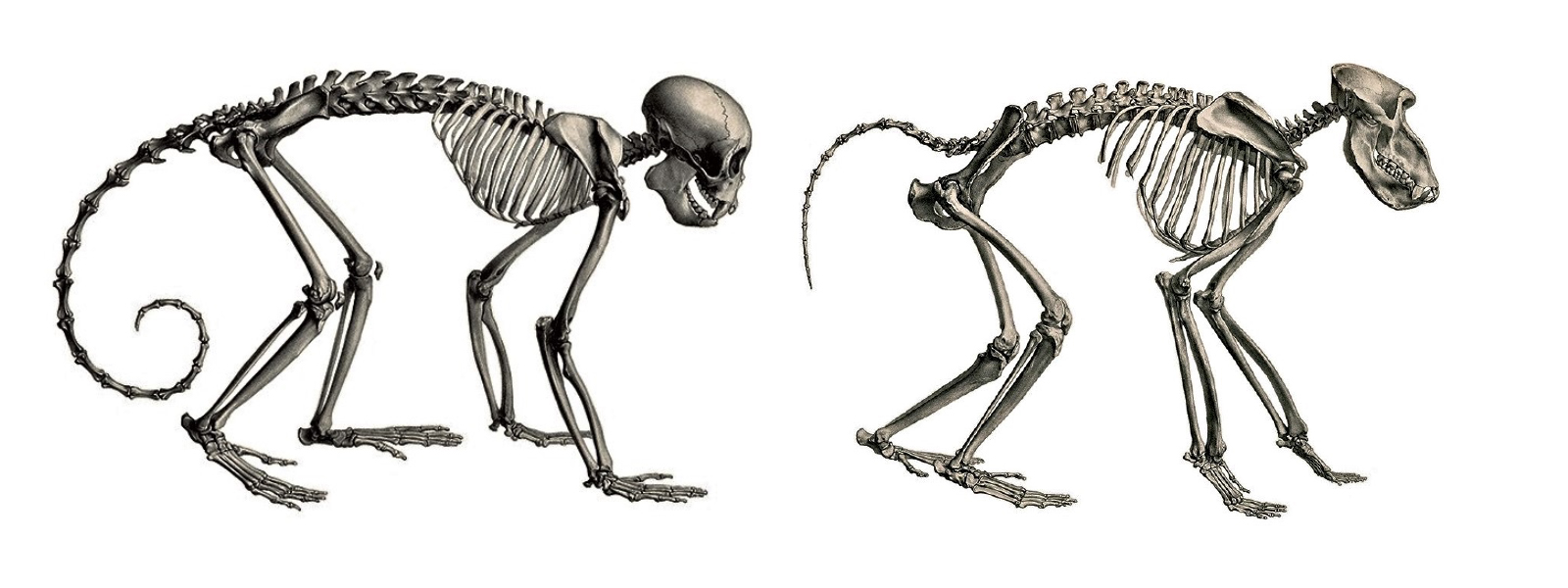
The third form of locomotion seen in primates is brachiation, the way of moving you used if you played on “monkey bars” as a child. Brachiation involves swinging below branches by the hands (Figure 5.12a). To be an efficient brachiator, a primate needs to have longer arms than legs, flexible shoulders and wrists, a short lower back, and no tail (Figure 5.12b). Some primates move via semi-brachiation, in which they swing below branches but do not have all of the same specializations as brachiators. Semi-brachiators have flexible shoulders, but their arms and legs are about the same length, which is useful because they are quadrupedal when on the ground. They also use long prehensile tails as a third limb when swinging (Figure 5.13). The underside of the tail has a tactile pad, resembling your fingerprints, for better grip.
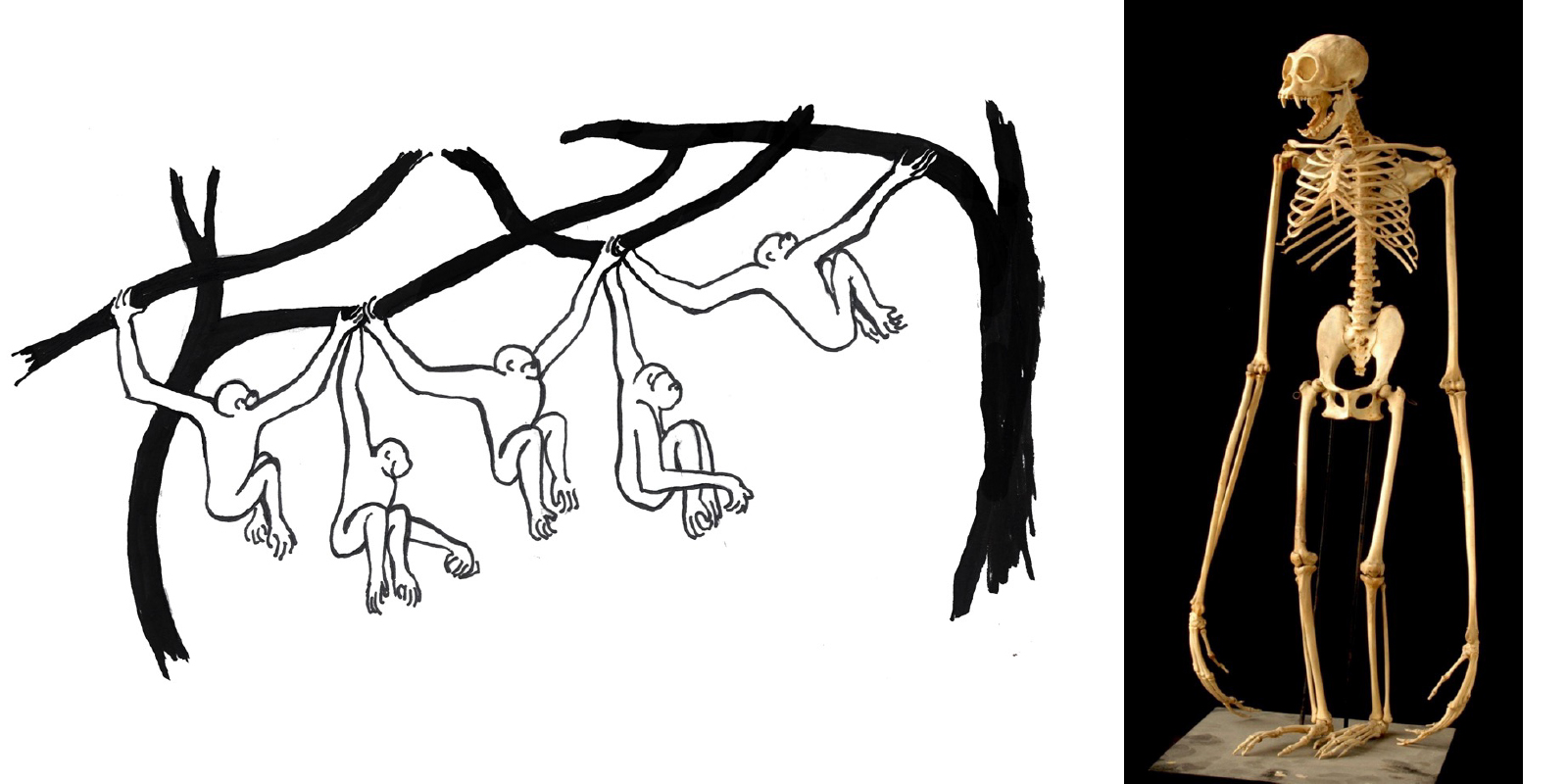
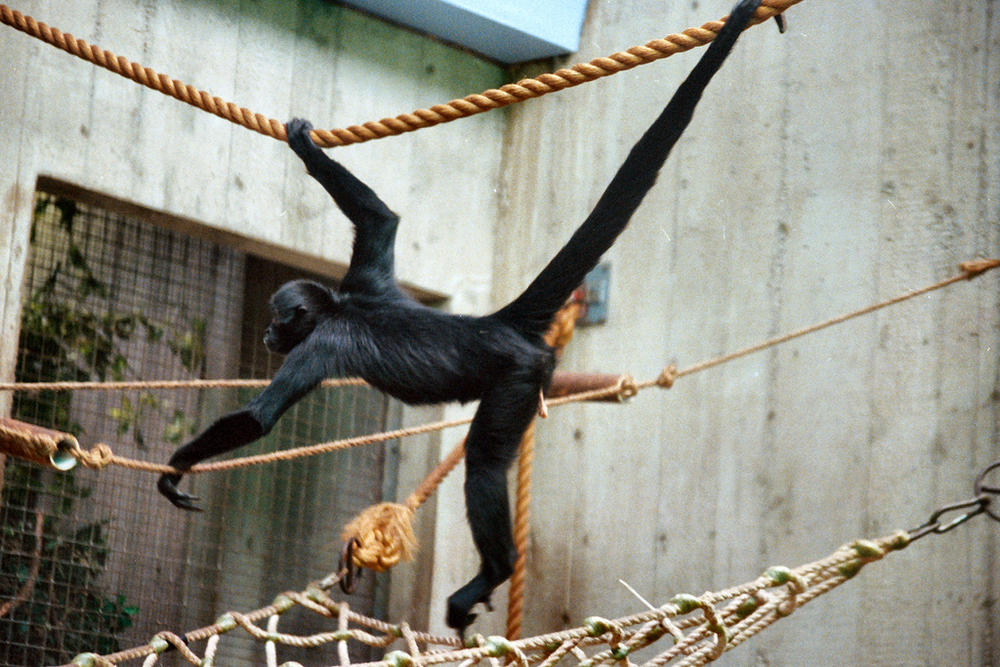
Lastly, humans move around on two feet, called bipedalism. Some nonhuman primates will occasionally travel on two feet but do so awkwardly and never for long distances. Among mammals, only humans have evolved to walk with a striding gait on two legs as a primary form of locomotion.
Primate Diversity
As we begin exploring the different taxa of primates, it is important to keep in mind the hierarchical nature of taxonomic classification and how this relates to the key characteristics that will be covered. Figure 5.14 summarizes the major taxonomic groups of primates that you will learn about here. If you locate humans on the chart, you can trace our classification and see all of the categories getting more inclusive as you work your way up to the Order Primates. This means that humans will have the key traits of each of those groups. It is a good idea to refer to the figure to orient yourself as we discuss each taxon.
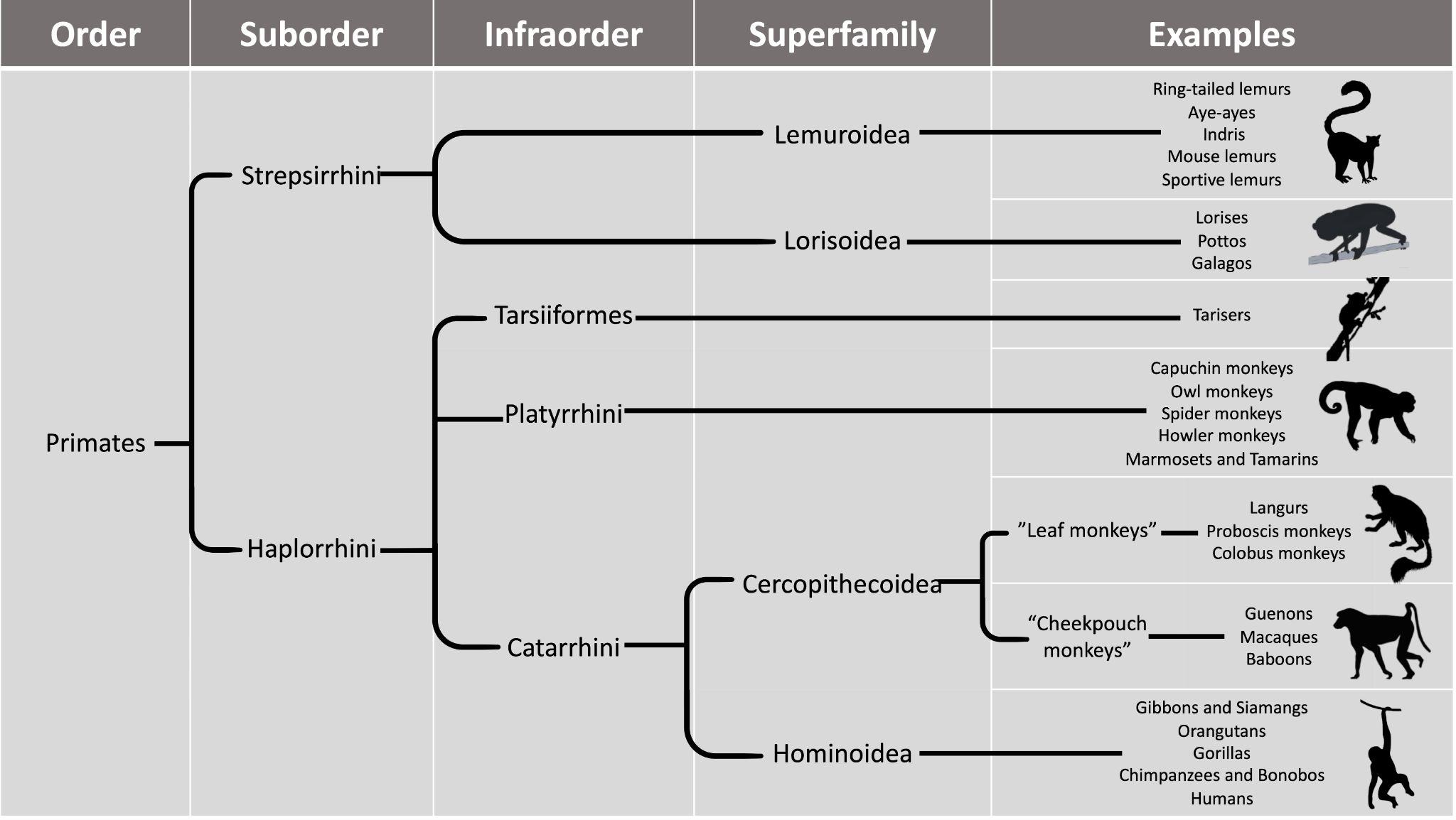
Suborder Strepsirrhini
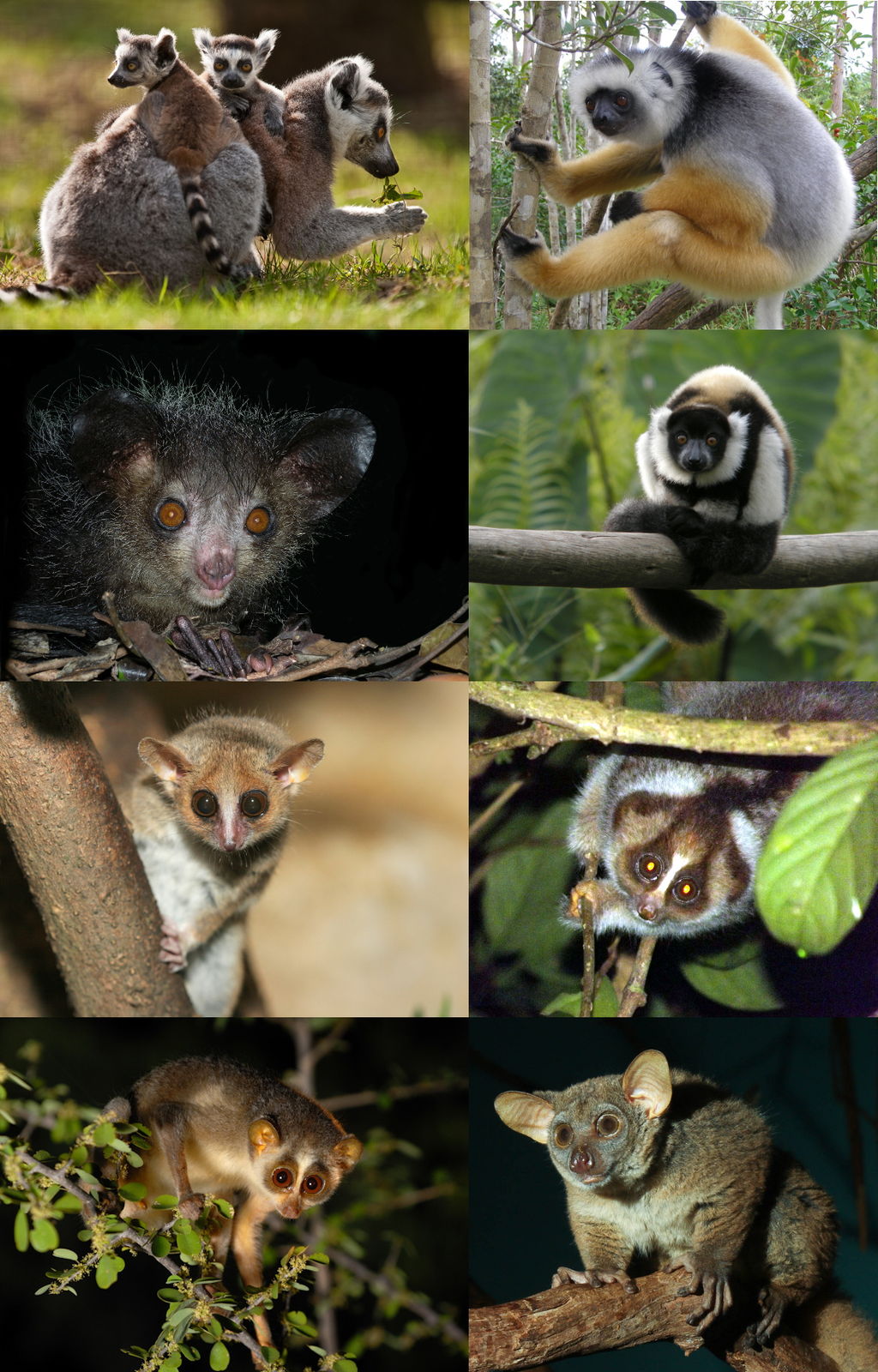
The Order Primates is subdivided into Suborder Strepsirrhini and Suborder Haplorrhini, which, according to molecular estimates, split about 70–80 million years ago (Pozzi et al. 2014). The strepsirrhines include the groups commonly called lemurs, lorises, and galagos (Figure 5.15). Strepsirrhines differ from haplorrhines in many ways, most of which involve retaining ancestral traits from the earliest primates. Strepsirrhines do have two key derived traits that evolved after they diverged from the haplorrhines: the grooming claw (Figure 5.16) on the second digit of each foot, and the tooth comb (or dental comb) located on the lower, front teeth (Figure 5.17). In most strepsirrhines, there are six teeth in the toothcomb—four incisors and two canines. Other than the tooth comb, the teeth of strepsirrhines are fairly simple and are neither large or distinctive relative to haplorrhines.
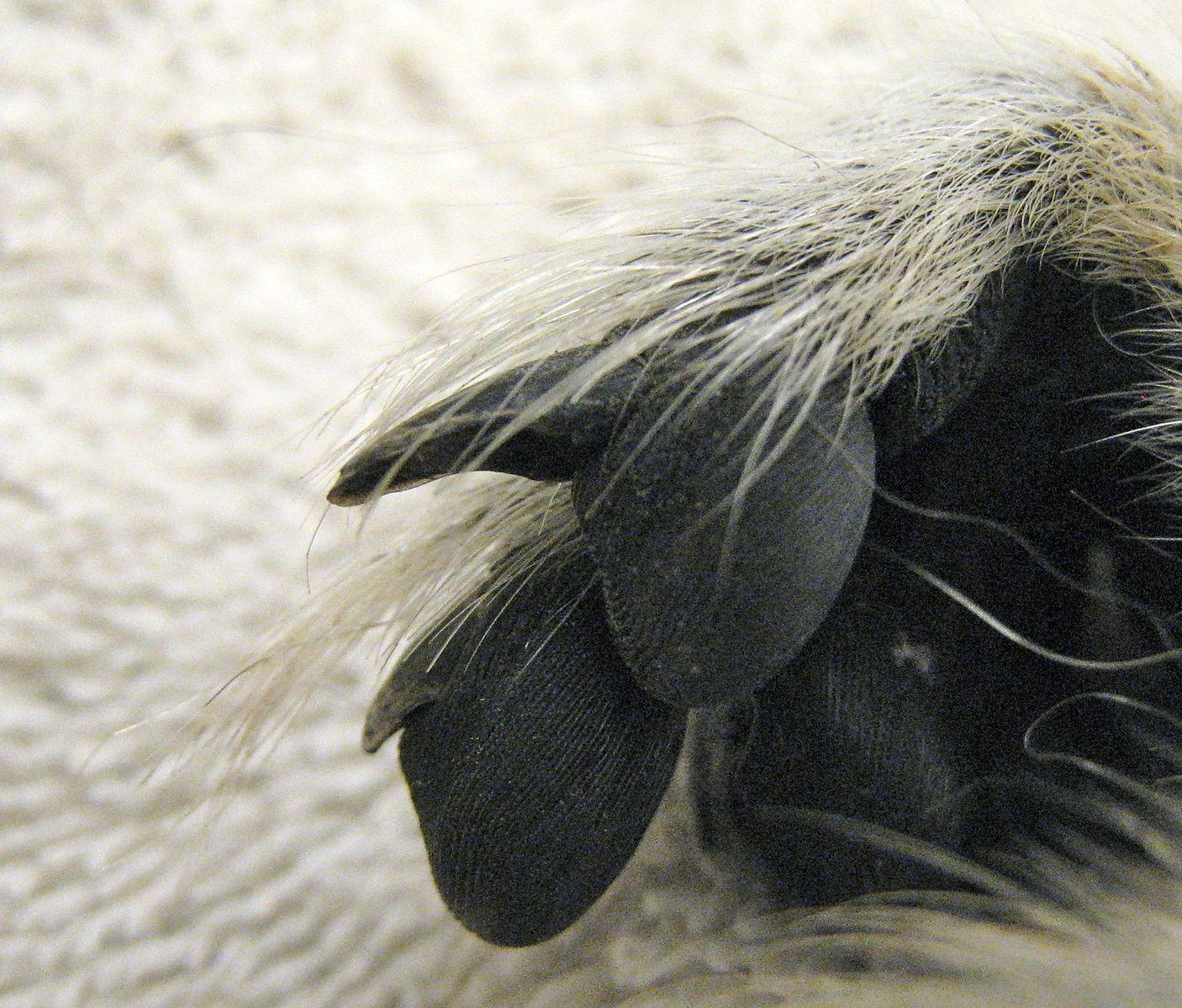
Compared to haplorrhines, strepsirrhines rely more on nonvisual senses. Strepsirrhines get their name because they have wet noses (rhinariums) like cats and dogs, a trait that, along with a longer snout, reflect strepsirrhines’ greater reliance on olfaction relative to haplorrhines. Many strepsirrhines use scent marking, including rubbing scent glands or urine on objects in the environment to communicate with others. Additionally, many strepsirrhines have mobile ears that they use to locate insect prey and predators. While strepsirrhines have a better sense of smell than haplorrhines, their visual adaptations are more ancestral. Strepsirrhines have less convergent eyes than haplorrhines and therefore all have postorbital bars, whereas haplorrhines have full postorbital closure (see Figure 5.2). All strepsirrhines have a tapetum lucidum, a reflective layer at the back of the eye that reflects light and thereby enhances the ability to see in low-light conditions. It is the same layer that causes your dog or cat to have “yellow eye” when you take photos of them with the flash on. This is a trait thought to be ancestral among mammals as a whole.
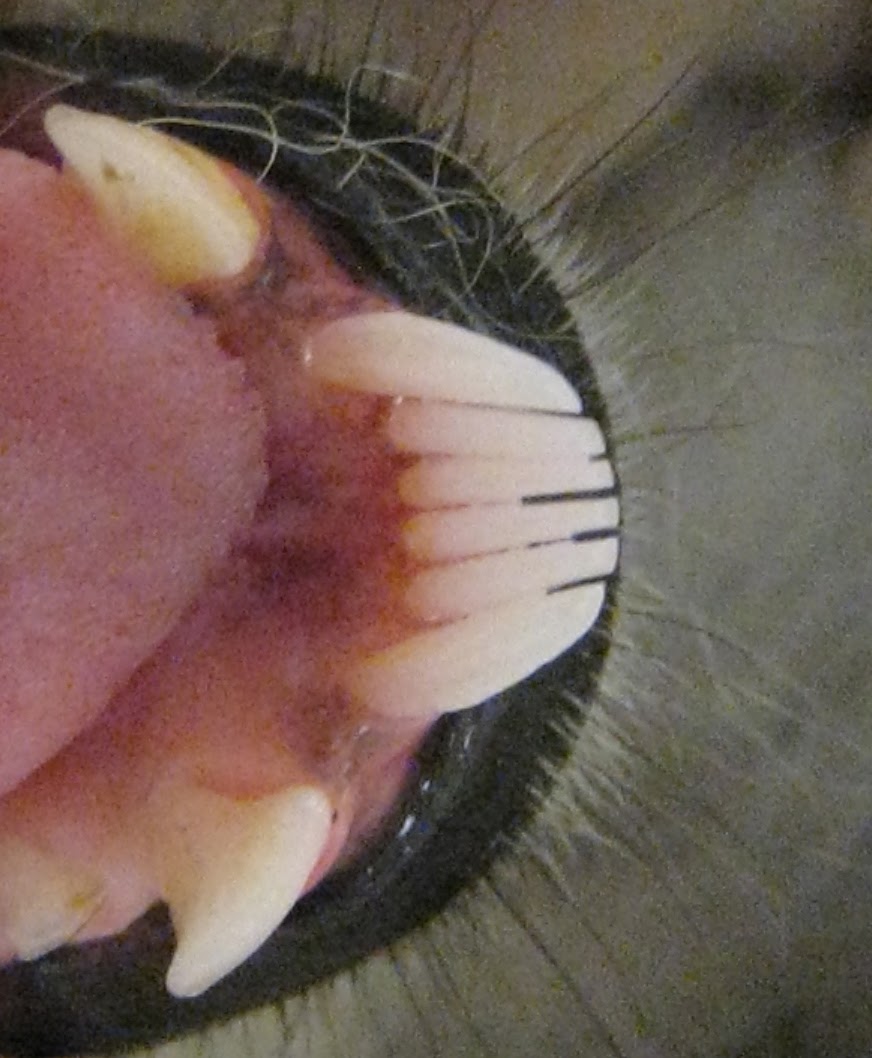
Strepsirrhines also differ from haplorrhines in some aspects of their ecology and behavior. The majority of strepsirrhines are solitary, traveling alone to search for food; a few taxa are more social. Most strepsirrhines are also nocturnal and arboreal. Strepsirrhines are, on average, smaller than haplorrhines, and so many of them have a diet consisting of insects and fruit, with few taxa eating primarily leaves. Lastly, most strepsirrhines are good at leaping, with several taxa specialized for vertical clinging and leaping. In fact, among primates, all but one of the vertical clinger leapers belong to the Suborder Strepsirrhini.
Strepsirrhines can be found all across Asia, Africa, and on the island of Madagascar (Figure 5.18). The Suborder Strepsirrhini is divided into two groups: (1) the lemurs of Madagascar and (2) the lorises, pottos, and galagos of Africa and Asia. By molecular estimates, these two groups split about 65 million years ago (Pozzi et al. 2014).
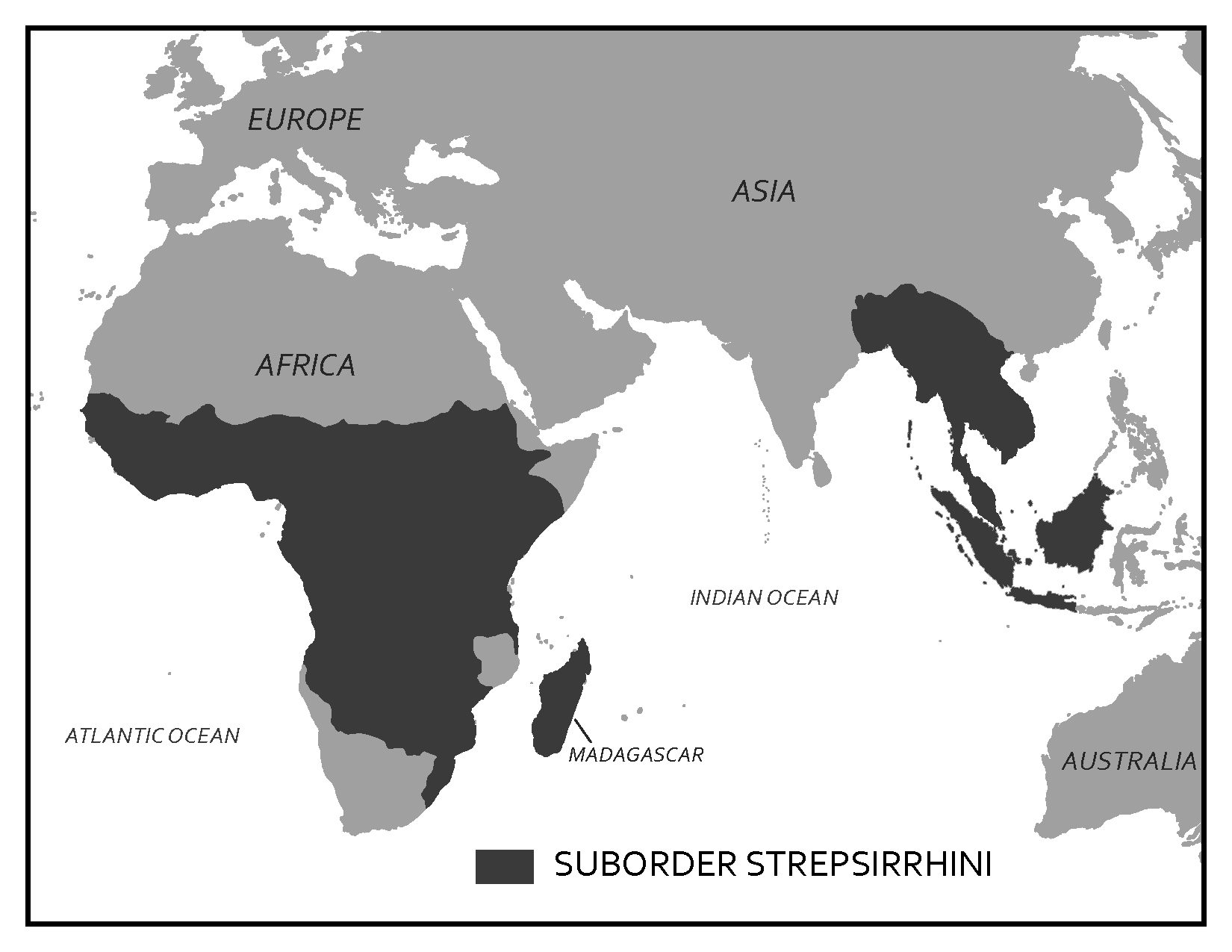
Lemurs of Madagascar
Madagascar is an island off the east coast of Africa, and it is roughly the size of California, Oregon, and Washington combined. It has been separated from Africa for about 130 million years and from India for about 85 million years, which means it was already an island when strepsirrhines got there approximately 60–70 million years ago. Only a few mammal species ever reached Madagascar, and so when lemurs arrived they were able to flourish into a variety of forms.
The lemurs of Madagascar are much more diverse compared to their mainland counterparts, the lorises and galagos. While many Malagasy strepsirrhines are nocturnal, plenty of others are diurnal or cathemeral. They range in body size from the smallest of all primates, the mouse lemur, some species of which weigh a little over an ounce (see Figure 5.15), up to the largest of all strepsirrhines, the indri, which weighs up to about 20 pounds (Figure 5.19). Lemurs include species that are insectivorous, frugivorous, and folivorous. A couple of members of this group have unusual diets for primates, including the gummivorous fork-marked and bamboo lemurs, who are able to metabolize the cyanide in bamboo. The most unique lemur is the aye-aye (depicted in Figure 5.15). This nocturnal lemur has rodent-like front teeth that grow continuously and a long-bony middle finger that it uses to fish grubs out of wood. It has a very large brain compared to other strepsirrhines, which it fuels with a diet that includes bird’s eggs and other animal matter. Based on genetic estimates and morphological studies, it is believed that aye-ayes were the first lemurs to separate from all other strepsirrhines and to evolve on their own since strepsirrhines arrived in Madagascar (Matsui et al. 2009).
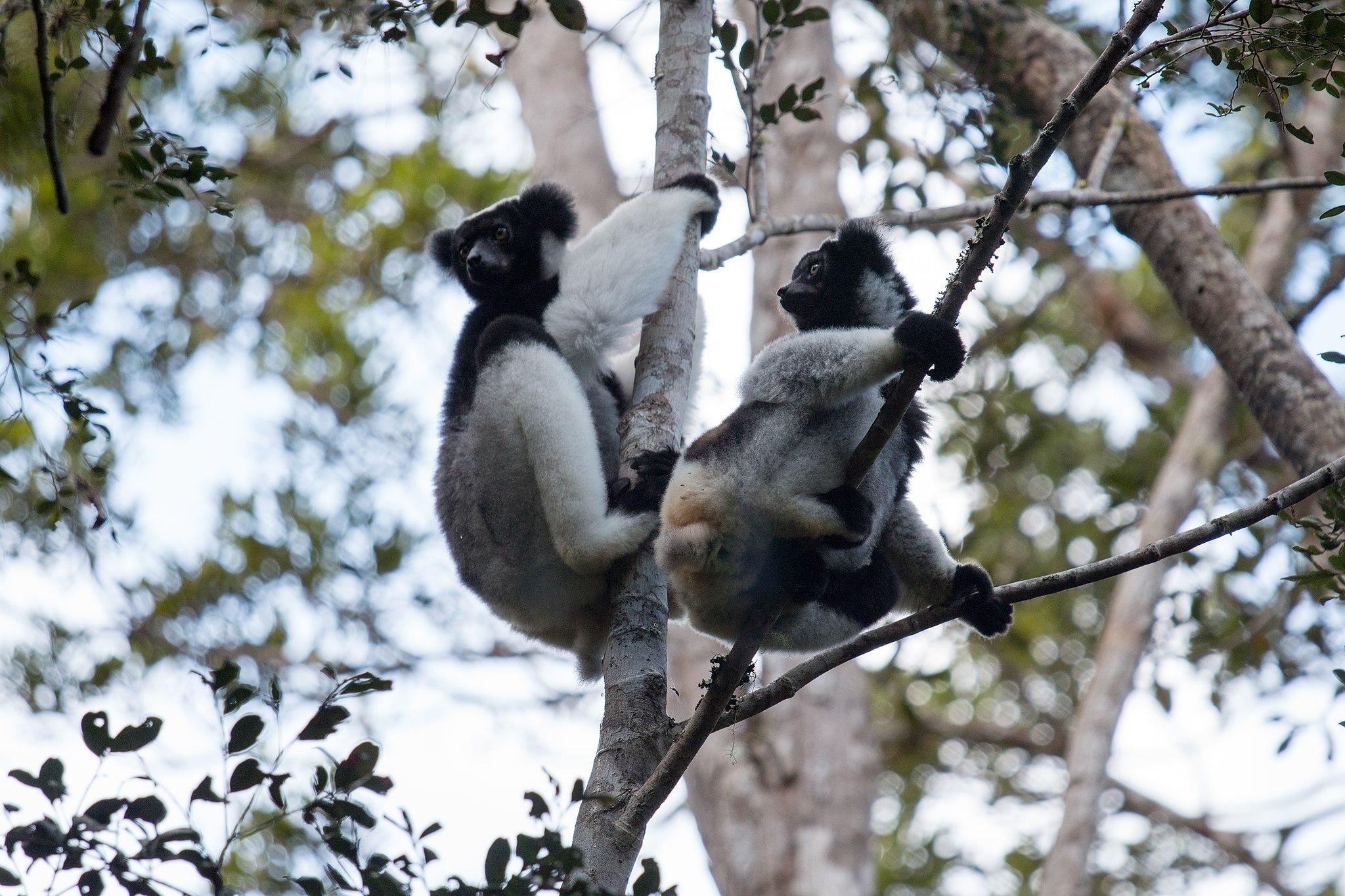
Lemurs are also diverse in terms of social behavior: Many lemurs are solitary foragers, some live in pairs, others in small groups, still others in larger groups, and some, like the red-ruffed lemur, live in unique and complex social groups (Vasey 2006). Lemurs include some of the best vertical clingers and leapers, and while many lemurs are quadrupedal, even the quadrupedal lemurs are quite adept at leaping. Malagasy strepsirrhines also exhibit a few unusual traits. They are highly seasonal breeders, often mating only during a short window once a year (Wright 1999). Female ring-tailed lemurs, for example, come into estrus one day a year for a mere six hours. Unlike most primates, where males are typically large and dominant, Malagasy strepsirrhines feature socially dominant females that are similar in size to males and have priority access to resources.
Lorises, Pottos, and Galagos of Asia and Africa
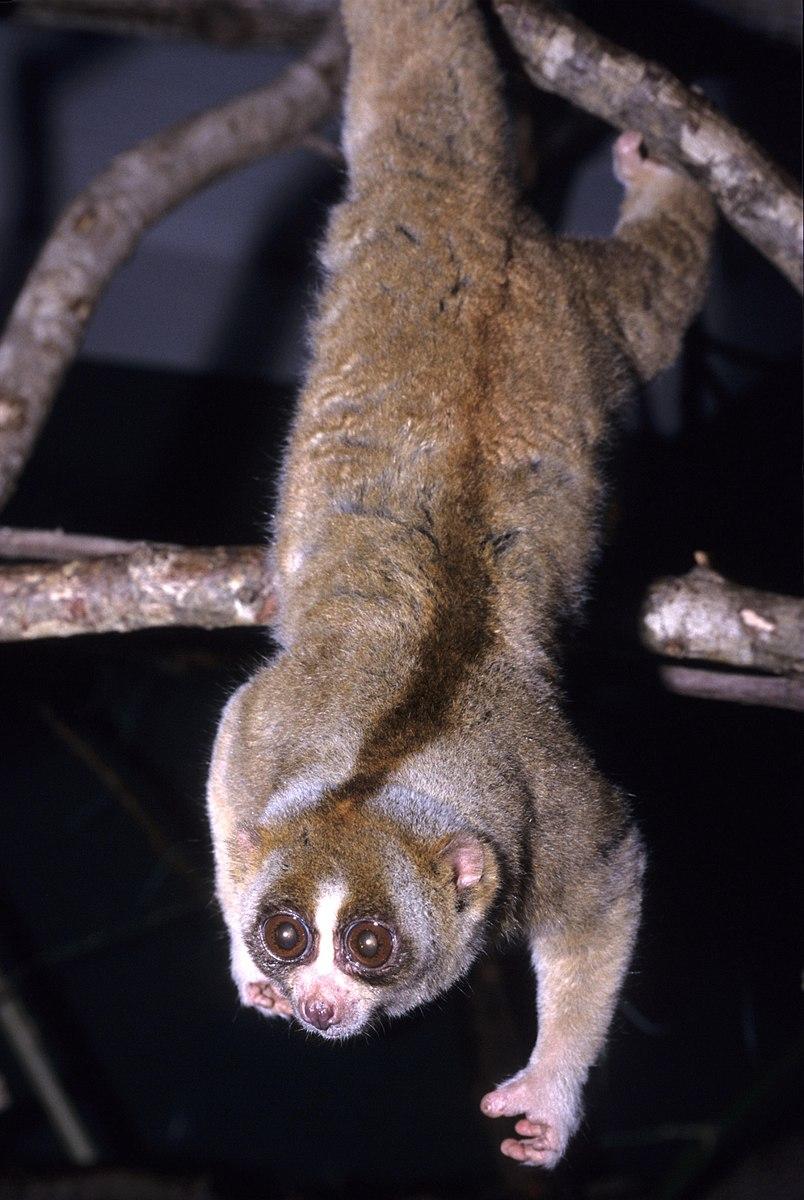
Unlike the lemurs of Madagascar, lorises, pottos, and galagos live in areas where they share their environments with monkeys and apes, who often eat similar foods. Lorises live across South and Southeast Asia, while pottos and galagos live across Central Africa. Because of competition with larger-bodied monkeys and apes, mainland strepsirrhines are more restricted in the niches they can fill in their environments and so are less diverse than the lemurs.
The strepsirrhines of Africa and Asia are all nocturnal and solitary, with little variation in body size and diet. For the most part, the diet of lorises, pottos, and galagos consists of fruits and insects. A couple of species eat more gum, but overall the diet of this group is narrow when compared to the Malagasy lemurs. Lorises (Figure 5.20) and pottos are known for being slow, quadrupedal climbers, moving quietly through the forests to avoid being detected by predators. These strepsirrhines have developed additional defenses against predators. Lorises, for example, eat a lot of caterpillars, which makes their saliva slightly toxic. Loris mothers bathe their young in this toxic saliva, making the babies unappealing to predators. In comparison to the slow-moving lorises and pottos, galagos are active quadrupedal runners and leapers that scurry about the forests at night. Galagos make distinctive calls that sound like a baby crying, which has led to their nickname “bushbabies.” Figure 5.21 summarizes the key differences between these two groups of strepsirrhines.
|
Lemurs |
Lorises, Pottos, and Galagos |
Geographic range |
Madagascar |
South and Southeast Asia Central Africa |
Activity patterns |
Diurnal, nocturnal, or cathemeral |
Nocturnal |
Dietary types |
Insectivore, frugivore, or folivore |
Insectivore, frugivore |
Social groupings |
Solitary, pairs, or small to large groups |
Solitary |
Forms of locomotion |
Vertical clinger leapers, quadrupedal |
Slow quadrupedal climbers and active quadrupedal runners |
Suborder Haplorrhini
When the two primate suborders split from one another, strepsirrhines retained more ancestral traits while haplorrhines developed more derived traits, which are discussed below.
As mentioned earlier, haplorrhines have better vision than strepsirrhines. This is demonstrated by the full postorbital closure protecting the more convergent eyes that haplorrhines possess (with one exception seen in Figure 5.2). Most haplorrhines are trichromatic, and all have a fovea, a depression in the retina at the back of the eye containing concentrations of cells that allows them to see things very close up in great detail. The heavier reliance on vision over olfaction is also reflected in the shorter snouts ending with the dry nose (no rhinarium) of haplorrhines. All but two genera of living haplorrhines are active during the day, so this group lacks the tapetum lucidum that is so useful to nocturnal species. On average, haplorrhines also have larger brains relative to their body size when compared with strepsirrhines.
The Haplorrhini differ from the Strepsirrhini in their ecology and behavior as well. Haplorrhines are generally larger than strepsirrhines, and they tend to be folivorous and frugivorous. This dietary difference is reflected in the teeth of haplorrhines, which are broader with more surface area for chewing. The larger body size of this taxon also influences locomotion. Only one haplorrhine is a vertical clinger and leaper. Most members of this suborder are quadrupedal, with one subgroup specialized for brachiation. A few haplorrhine taxa are monomorphic, meaning males and females are the same size, but many members of this group show moderate to high sexual dimorphism in body size and canine size. Haplorrhines also differ in social behavior. All but two haplorrhines live in groups, which is very different from the primarily solitary strepsirrhines. Differences between the two suborders are summarized in Figure 5.22.
|
|
Suborder Haplorrhini |
Sensory adaptations |
Rhinarium Longer snout Eyes less convergent Postorbital bar Tapetum lucidum Mobile ears |
No rhinarium Short snout Eyes more convergent Postorbital plate No tapetum lucidum Many are trichromatic Fovea |
Dietary differences |
Mostly insectivores and frugivores, few folivores |
Few insectivores, mostly frugivores and folivores |
Activity patterns and Ecology |
Mostly nocturnal, few diurnal or cathemeral Almost entirely arboreal |
Only two are nocturnal, rest are diurnal Many arboreal taxa, also many terrestrial taxa |
Social groupings |
Mostly solitary, some pairs, small to large groups |
Only two are solitary, all others live in pairs, small to very large groups |
Sexual dimorphism |
Minimal to none |
Few taxa have little/none, many taxa show moderate to high dimorphism |
Suborder Haplorrhini is divided into three infraorders: Tarsiiformes, which includes the tarsiers of Asia; Platyrrhini, which includes the monkeys of Central and South America; and Catarrhini, a group that includes the monkeys of Asia and Africa, apes, and humans. According to molecular estimates, tarsiers split from the other haplorrhines close to 70 million years ago, and platyrrhines split from catarrhines close to 46 million years ago (Pozzi et al. 2014).
Infraorder Tarsiiformes of Asia
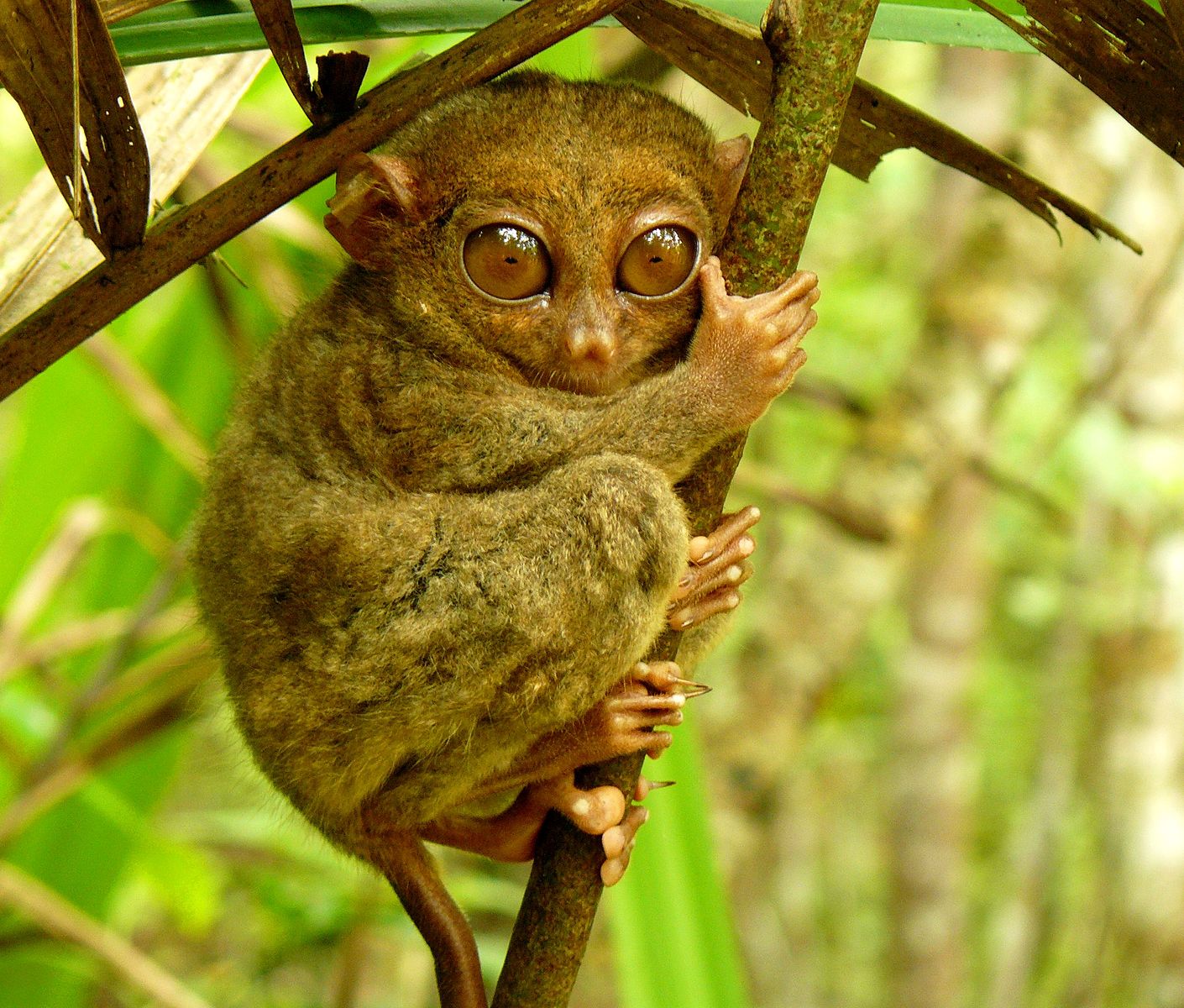
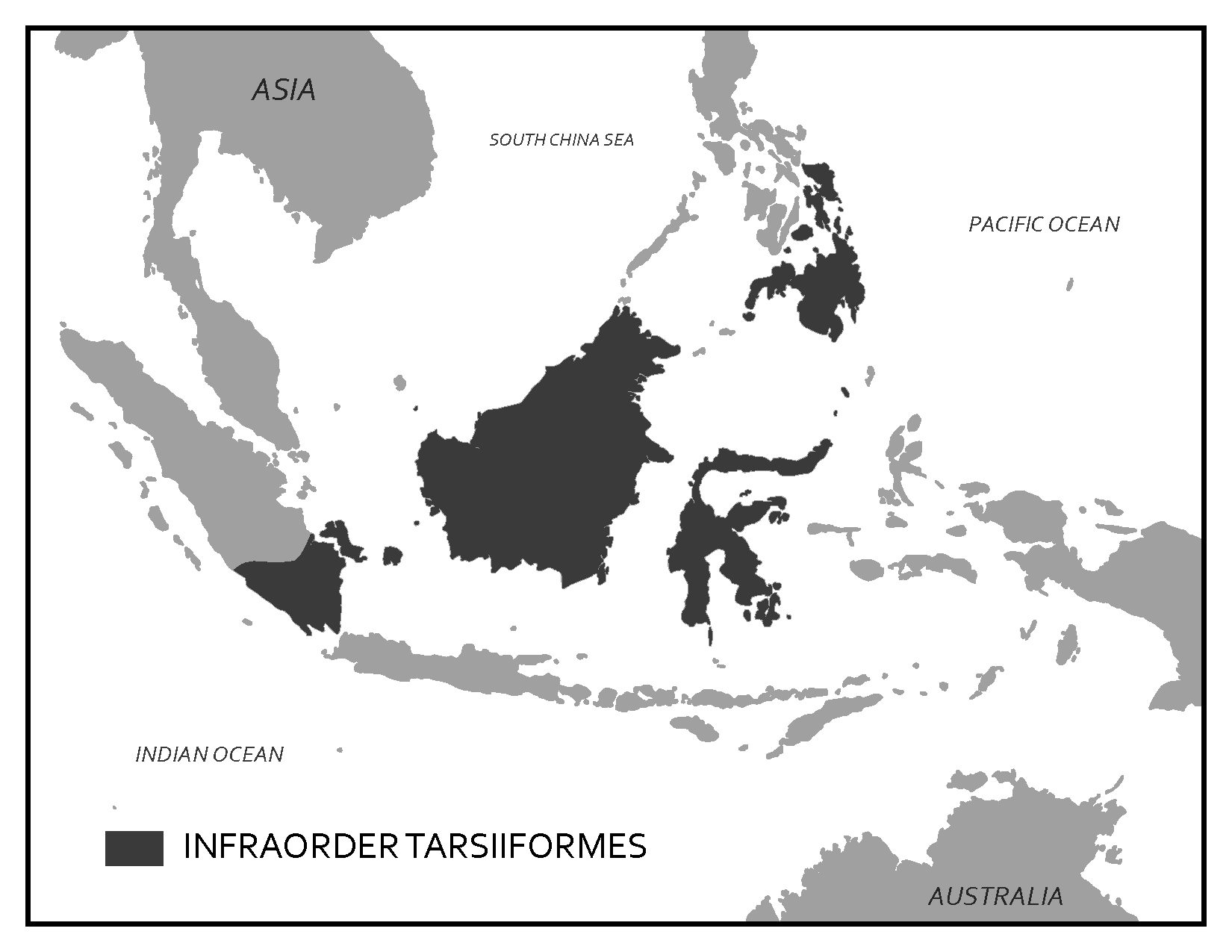
Today, the Infraorder Tarsiiformes includes only one genus, Tarsius (Figure 5.23). Tarsiers are small-bodied primates that live in Southeast Asian forests (Figure 5.24) and possess an unusual collection of traits that have led to some debate about their position in the primate taxonomy. They are widely considered members of the haplorrhine group because they share several derived traits with monkeys, apes, and humans, including dry noses, a fovea, not having a tapetum lucidum, and eyes that are more convergent. Tarsiers also have some traits that are more like strepsirrhines and some that are unique. Tarsiers are the only haplorrhine that are specialized vertical clinger leapers, a form of locomotion only otherwise seen in some strepsirrhines. Tarsiers actually get their name because their ankle (tarsal) bones are elongated to provide a lever for vertical clinging and leaping. Tarsiiformes are also small, with most species weighing between 100 and 150 grams. Like strepsirrhines, tarsiers are nocturnal, but because they lack a tapetum lucidum, tarsiers compensate by having enormous eyes. In fact, each eye of a tarsier is larger than its brain. These large eyes allow enough light in for tarsiers to still be able to see well at night without the reflecting layer in their eyes. To protect their large eyes, tarsiers have a partially closed postorbital plate that appears somewhat intermediate between the postorbital bar of strepsirrhines and the full postorbital closure of other haplorrhines (Figure 5.25). Tarsiers have different dental formulas on their upper and lower teeth. On the top, the dental formula is 2:1:3:3, but on the bottom it is 1:1:3:3. Other unusual traits of tarsiers include having two grooming claws on each foot and the ability to rotate their heads around 180 degrees, a trait useful in locating insect prey. The tarsier diet is considered faunivorous because it consists entirely of animal matter, making them the only primate not to eat any vegetation. They are only one of two living haplorrhines to be solitary, the other being the orangutan. Most tarsiers are not sexually dimorphic, like strepsirrhines, although males of a few species are slightly larger than females.
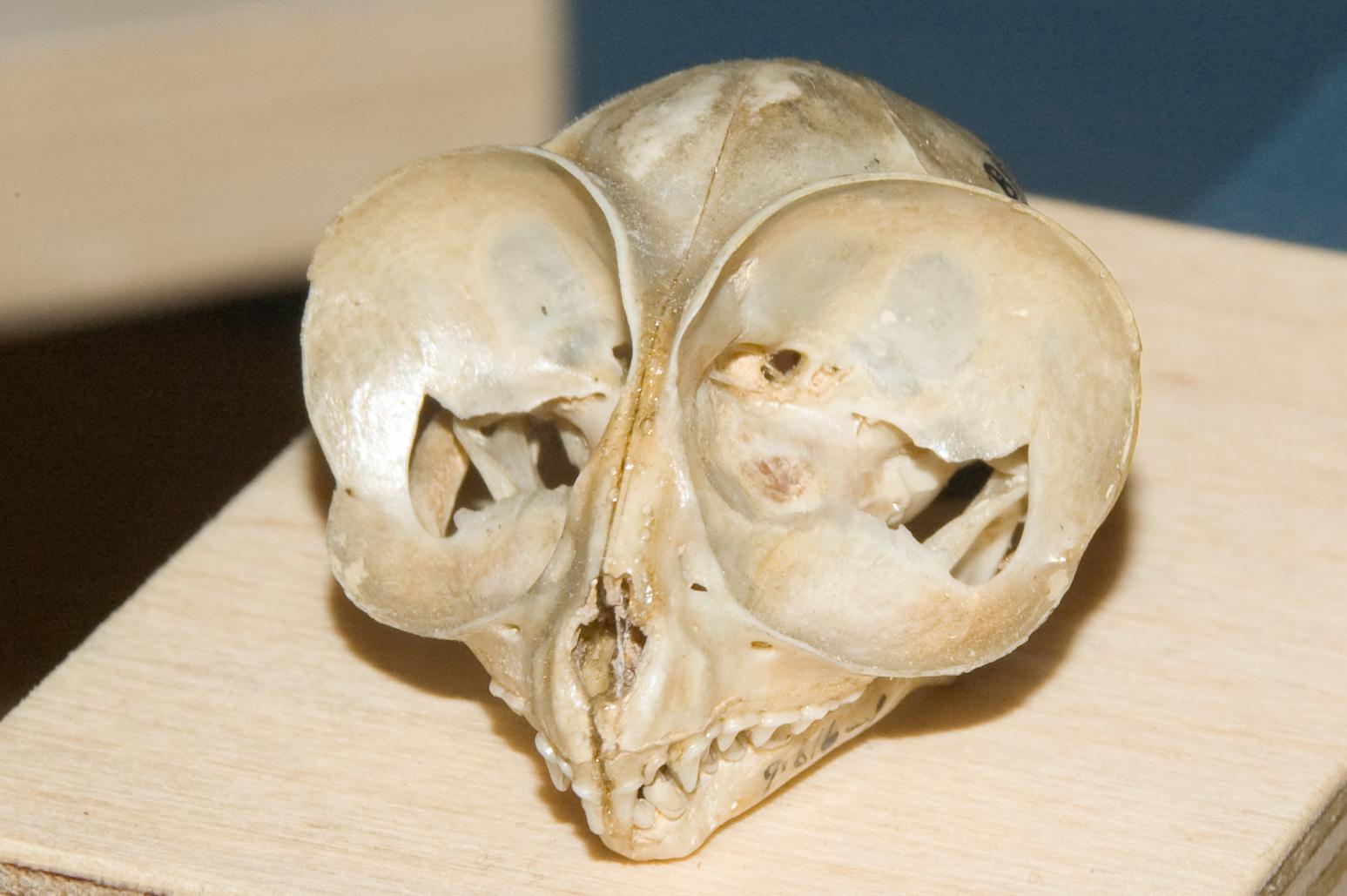
Two alternative classifications have emerged due to the unusual mix of traits that tarsiers have. Historically, tarsiers were grouped with lemurs, lorises, and galagos into a suborder called Prosimii. This classification was based on tarsiers, lemurs, lorises, and galagos all having grooming claws and similar lifestyles. Monkeys, apes, and humans were then separated into a suborder called the Anthropoidea. These suborder groupings were based on grade rather than clade. Today, most people use Suborders Strepsirrhini and Haplorrhini, which are clade groupings based on the derived traits that tarsiers share with monkeys, apes, and humans. The Strepsirrhini/Haplorrhini dichotomy is also supported by the genetic evidence that indicates tarsiers are more closely related to monkeys, apes, and humans (Jameson et al. 2011). Figure 5.26 summarizes the unusual mix of traits seen in tarsiers.
Like Strepsirrhini |
Unique |
Like Haplorrhini |
Very small Nocturnal Highly insectivorous Solitary Vertical clinger-leapers Little/no sexual dimorphism |
Two grooming claws 2:1:3:3/1:1:3:3 dental formula Do not eat vegetation Can rotate their heads nearly 180 degrees |
Almost full PO closure More convergent eyes No tapetum lucidum No rhinarium Genetic evidence Fovea |
Infraorder Platyrrhini of Central and South America
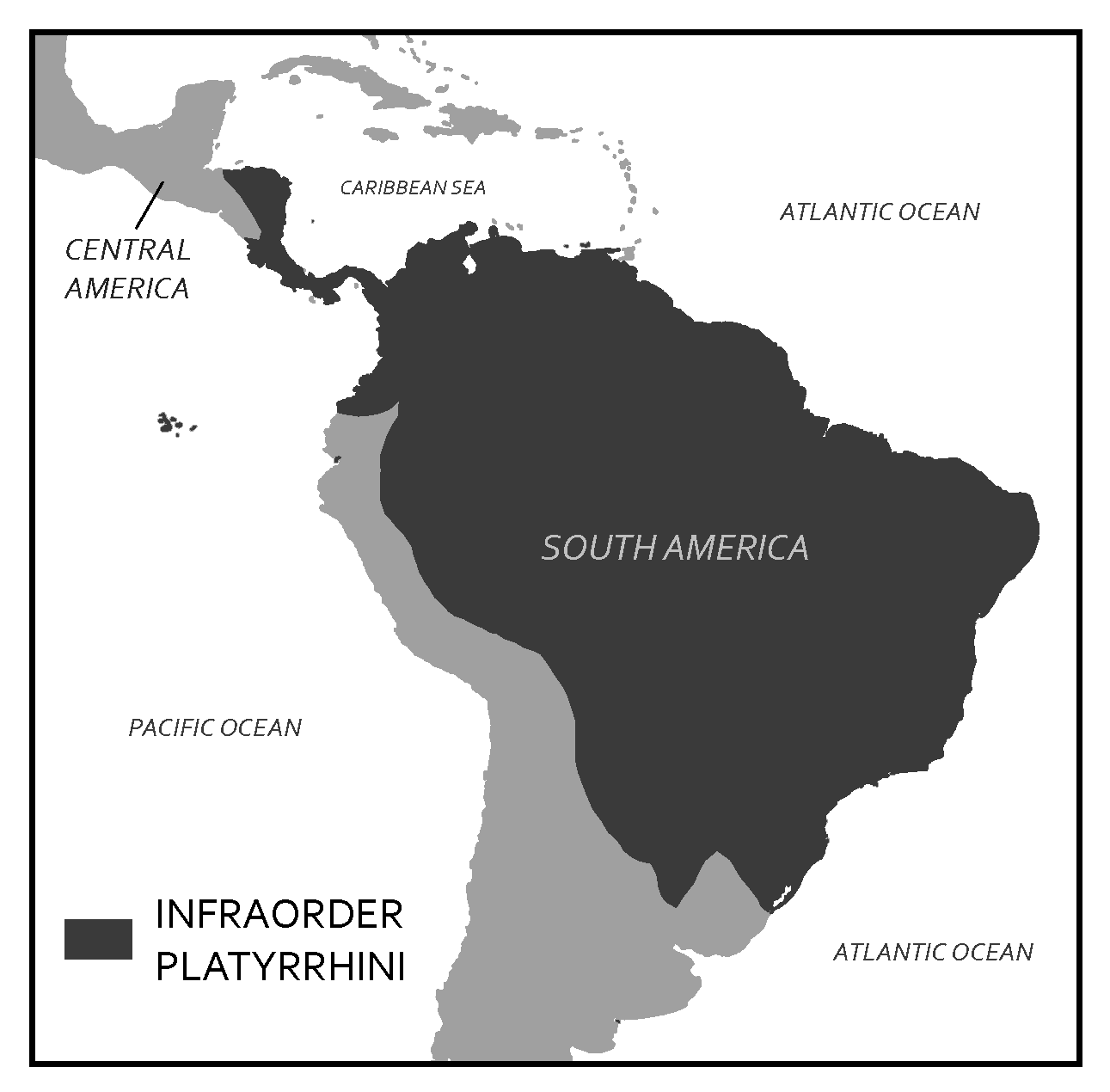
The platyrrhines are the only nonhuman primates in Central and South America (Figure 5.27) and so, like the lemurs of Madagascar, have diversified into a variety of forms in the absence of competition. Infraorder Platyrrhini get their name from their distinctive nose shape. “Platy” means flat and “rhini” refers to noses, and, indeed, platyrrhines have noses that are flat and wide, with nostrils that are far apart, facing outward, and usually round in shape (Figure 5.28). This nose shape is very different from what we see in catarrhines.
On average, platyrrhines are smaller and less sexually dimorphic than catarrhines, and they have retained the more ancestral primate dental formula of 2:1:3:3. Platyrrhines are all highly arboreal, whereas many catarrhines spend significant time on the ground. The monkeys in Central and South America also differ in having less well-developed vision. This is reflected in the wiring in the visual system of the brain as well as in their polymorphic color vision. The genes that enable individuals to distinguish reds and yellows from blues and greens are on the X chromosome. Different genes code for being able to see different wavelengths of light so to distinguish between them you need to be heterozygous for seeing color. The X chromosomes of platyrrhines each carry the genes for seeing one wavelength, so male platyrrhines (with only one X chromosome) are always dichromatic. Female platyrrhines can be dichromatic (if they are homozygous for one version of the color vision gene) or trichromatic (if they are heterozygous) (Kawamura et al. 2012). We currently know of two exceptions to this pattern among platyrrhines. Nocturnal owl monkeys are monochromatic, meaning that they cannot distinguish any colors. The other exception are howler monkeys, which have evolved to have two color vision genes on each X chromosome. This means that both male and female howler monkeys are able to see reds and yellows. By contrast, catarrhine males and females are all trichromatic.
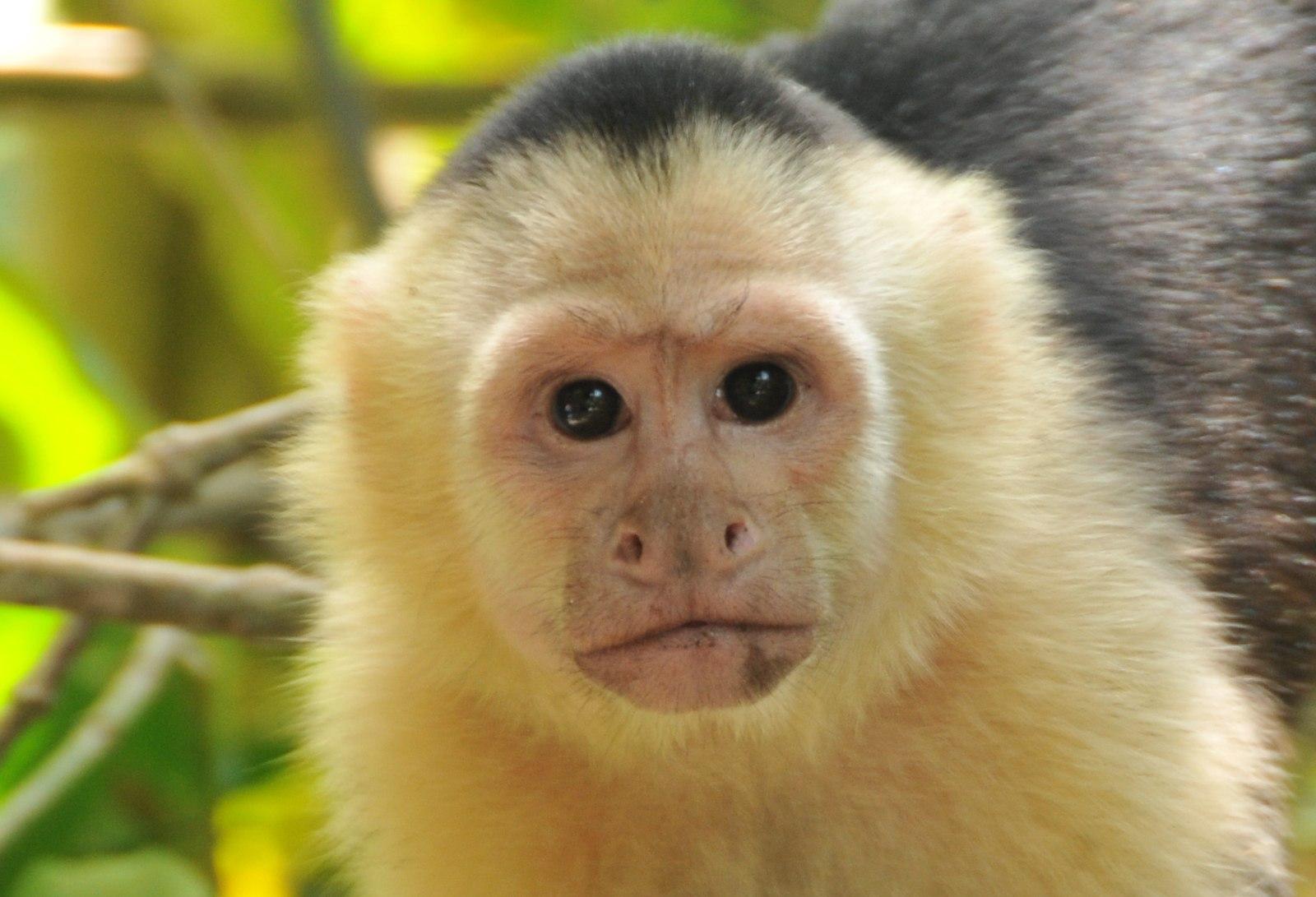
Platyrrhines include the smallest of the monkeys, the marmosets and tamarins (Figure 5.29), all of which weigh less than one kilogram and live in cooperative family groups, wherein usually only one female reproduces and everyone else helps carry and raise the offspring. They are unusual primates in that they regularly produce twins. Marmosets and tamarins largely eat gums and saps, so these monkeys have evolved claw-like nails that enable them to cling to the sides of tree trunks like squirrels as well as special teeth that allow them to gnaw through bark. Except for the Goeldi’s monkey, these small monkeys have one fewer molar than other platyrrhines, giving them a dental formula of 2:1:3:2.
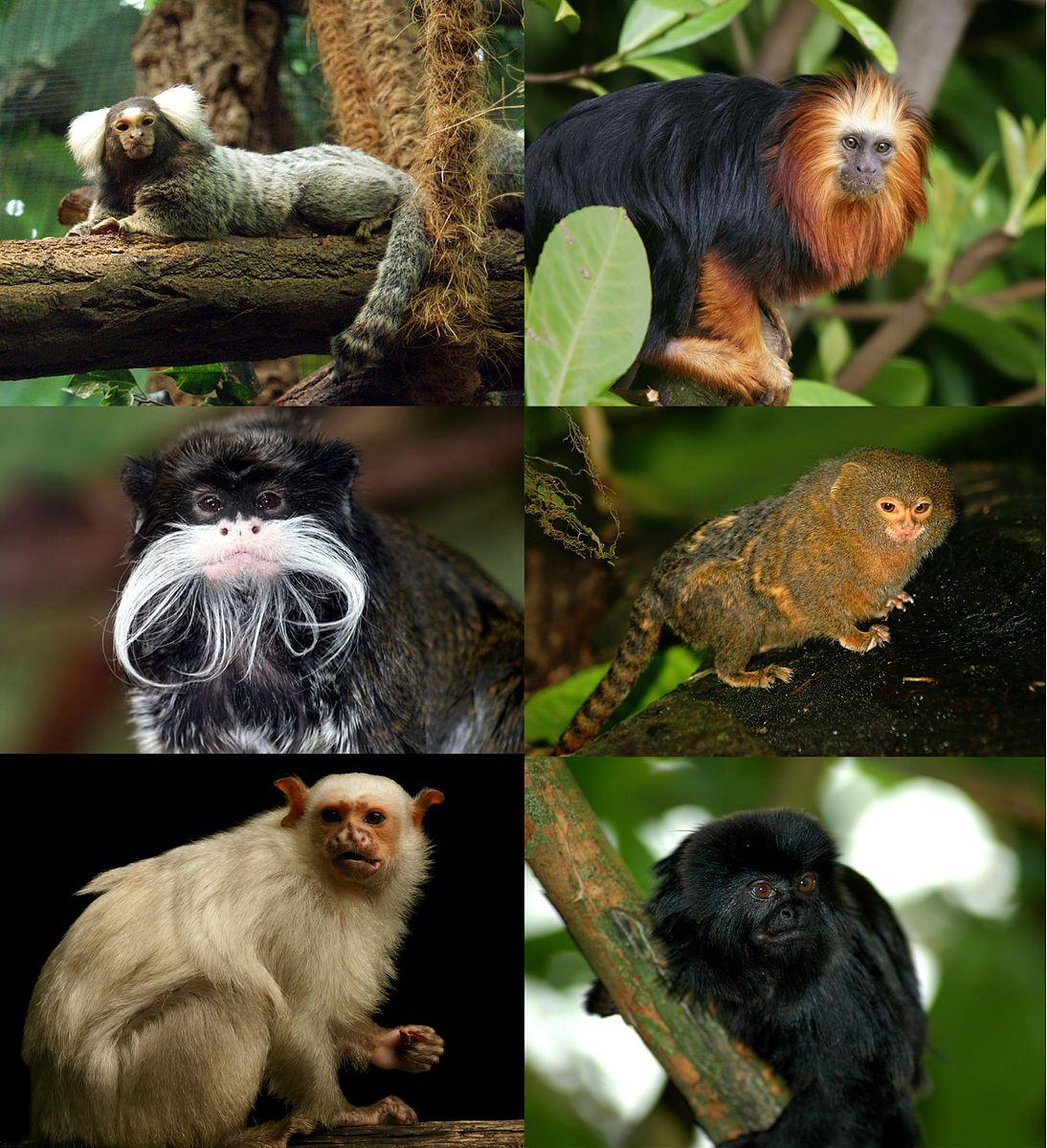
The largest platyrrhines are a family that include spider monkeys, woolly spider monkeys, woolly monkeys, and howler monkeys (Figure 5.30). These monkeys can weigh up to 9–15 kg and have evolved prehensile tails that can hold their entire body weight. It is among this group that we see semi-brachiators, like the spider monkey (see Figure 5.13). To make them more efficient in this form of locomotion, spider monkeys evolved to not have thumbs so that their hands work more like hooks that can easily let go of branches while swinging. Howler monkeys are another well-known member of this group, earning their name due to their loud calls, which can be heard miles away. To make these loud vocalizations, howler monkeys have a specialized vocal system that includes a large larynx and hyoid bone. Howler monkeys are the most folivorous of the platyrrhines and are known for spending a large portion of their day digesting their food.
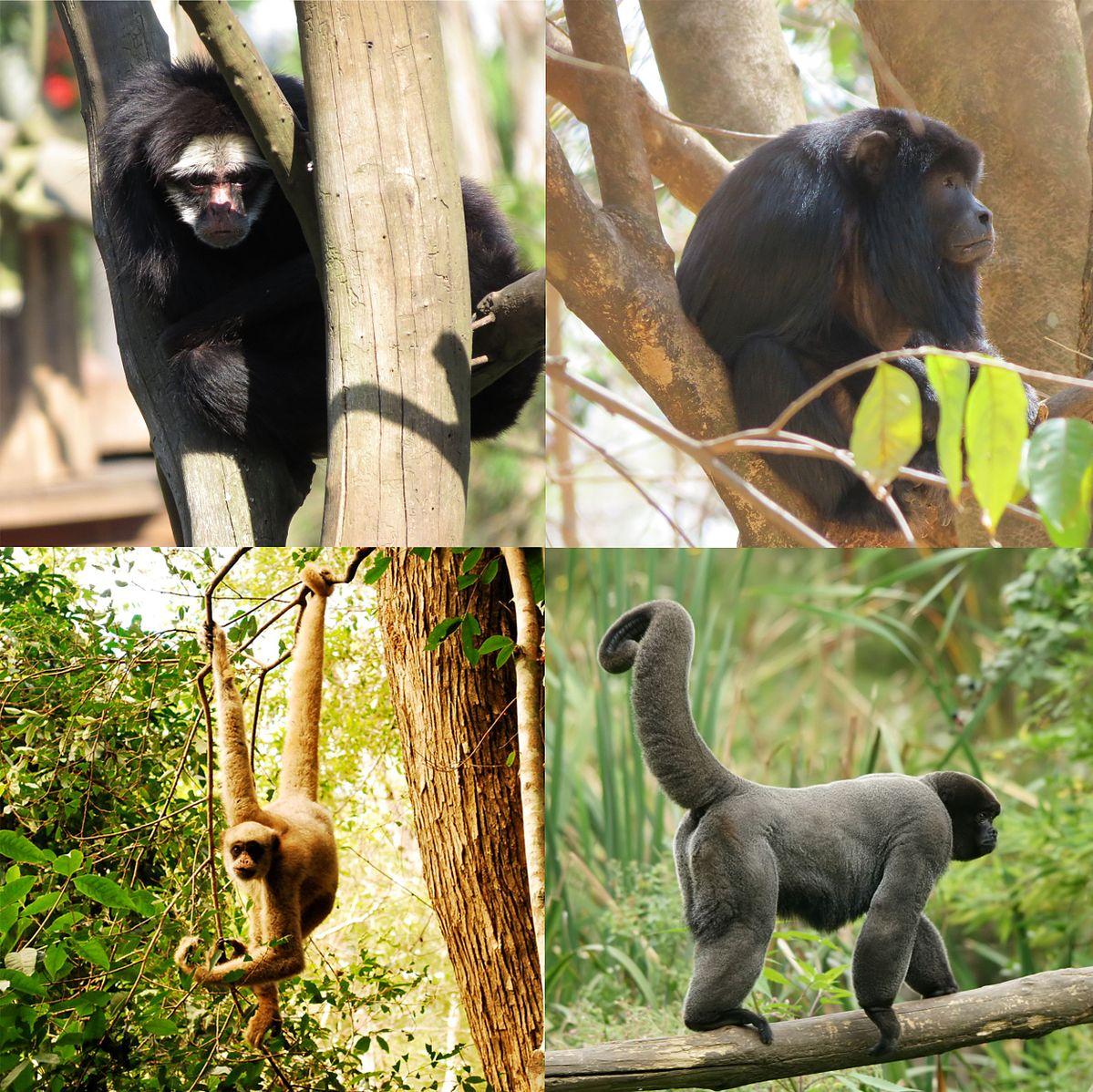
There are many other monkeys in Central and South America, including the gregarious capuchins (see Figure 5.28) and squirrel monkeys, the pair-living titi monkeys, and the nocturnal owl monkeys. There are also the seed-eating saki monkeys and uakaris. In many areas across Central and South America, multiple species of platyrrhines share the forests, with some even traveling together in association. According to molecular evidence, the diversity of platyrrhines that we see today seems to have originated about 25 million years ago (Schneider and Sampaio 2015). Figure 5.31 summarizes the key traits of platyrrhines relative to the other infraorders of Haplorrhini.
Platyrrhini traits |
Flat nose with rounded nostrils pointing to the side Highly arboreal Less sexually dimorphic on average 2:1:3:3 dental formula* Polymorphic color vision* |
Infraorder Catarrhini of Asia and Africa
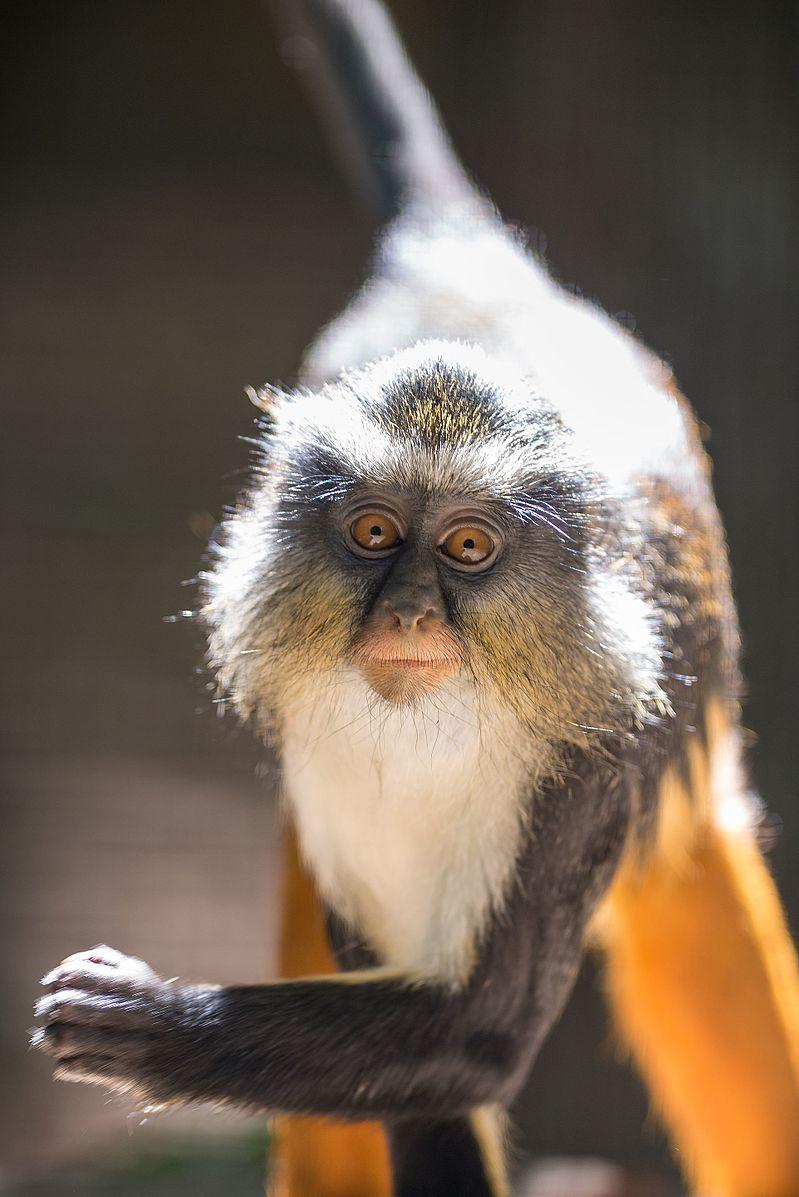
Infraorder Catarrhini includes Superfamily Cercopithecoidea (the monkeys of Africa and Asia) and Superfamily Hominoidea (apes and humans). Nonhuman catarrhines are found all over Africa and South and Southeast Asia, with some being found as far north as Japan. The most northerly and southerly catarrhines are cercopithecoid monkeys. In contrast, apes are less tolerant of drier, more seasonal environments and so have a relatively restricted geographic range.
Relative to other haplorrhine infraorders, catarrhines are distinguished by several characteristics. Catarrhines have a distinctive nose shape, with teardrop-shaped nostrils that are close together and point downward (Figure 5.32) and one fewer premolar than most other primates, giving us a dental formula of 2:1:2:3 (Figure 5.33). On average, catarrhines are the largest and most sexually dimorphic of all primates. Gorillas are the largest living primates, with males weighing up to 220 kg. The most sexually dimorphic of all primates are mandrills. Mandrill males not only have much more vibrant coloration than mandrill females but also have larger canines and can weigh up to three times more (Setchell et al. 2001). The larger body size of catarrhines is related to the more terrestrial lifestyle of many members of this infraorder. In fact, the most terrestrial of living primates can be found in this group. Among all primates, vision is the most developed in catarrhines. Catarrhines independently evolved the same adaptation as howler monkeys in having each X chromosome with genes to distinguish both reds and yellows, so all male and female catarrhines are trichromatic, which is useful for these diurnal primates.
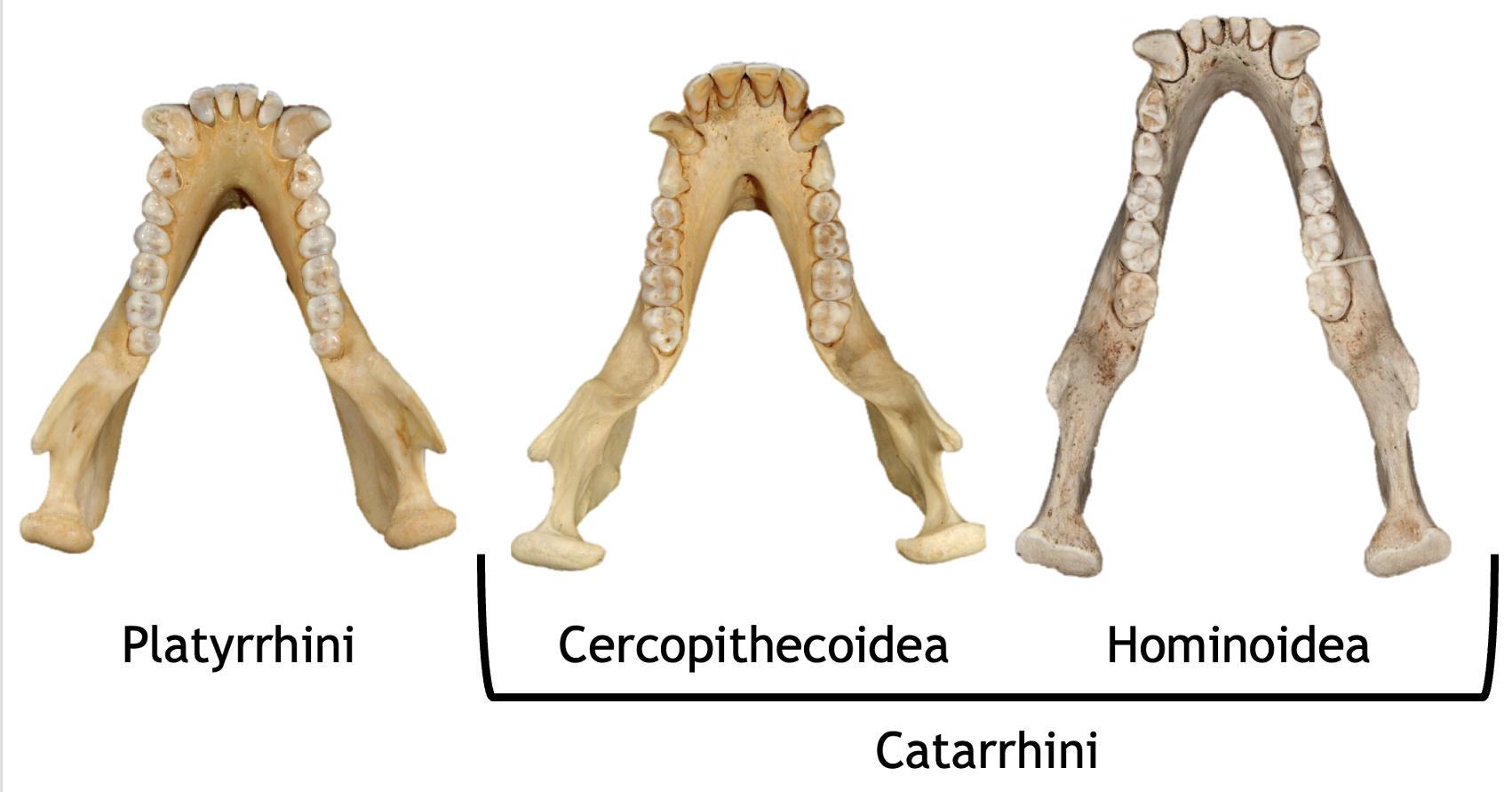
The two superfamilies of catarrhines—Superfamily Cercopithecoidea, the monkeys of Africa and Asia, and Superfamily Hominoidea, which includes apes and humans—are believed to have split about 32 million years ago based on molecular evidence (Pozzi et al. 2014). This fits with the fossil record, which shows evidence of these lineages by about 25 million years ago (see Chapter 8).
Superfamily Cercopithecoidea of Africa and Asia
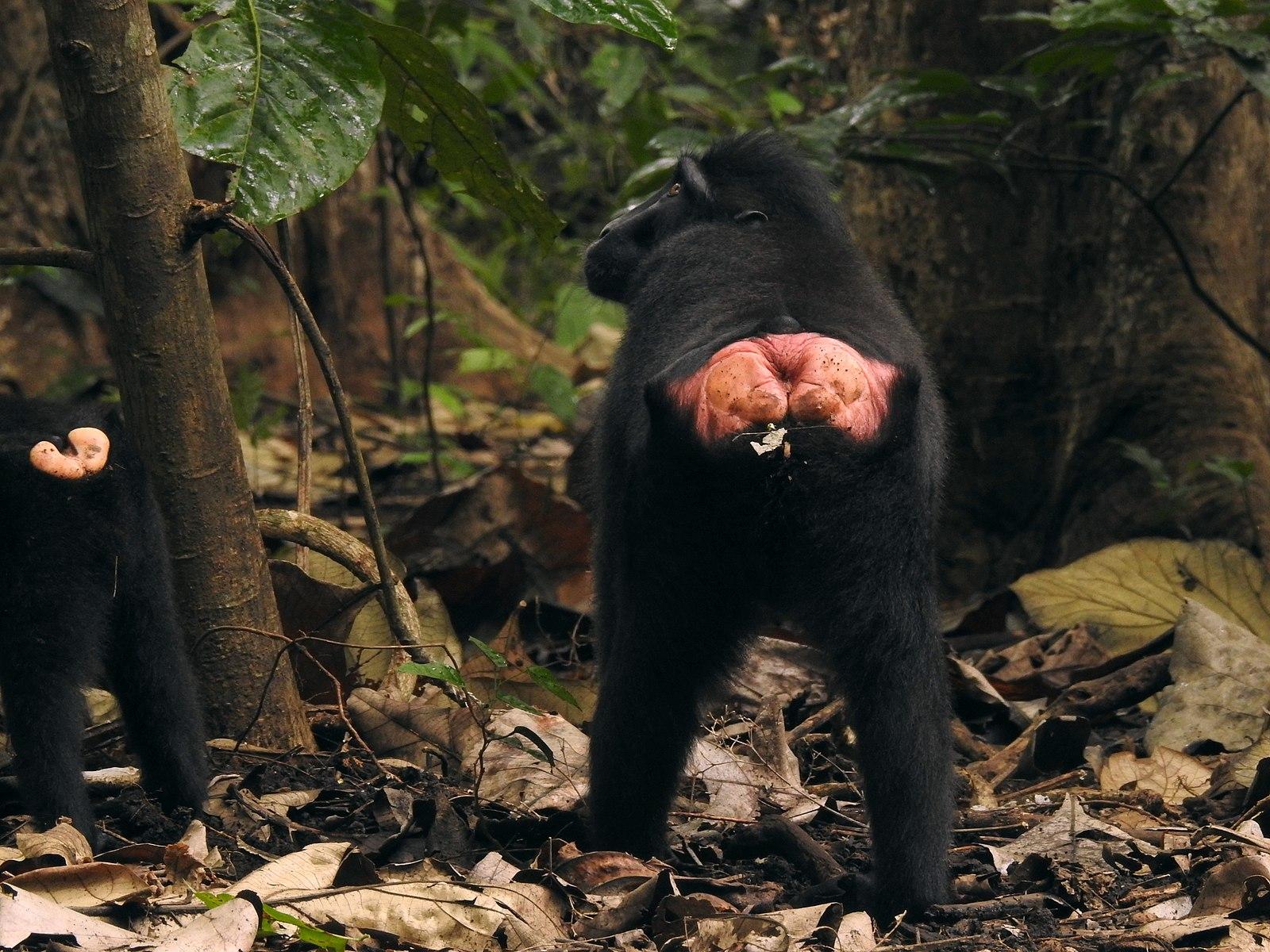
Compared to hominoids, cercopithecoids have an ancestral quadrupedal body plan with two key derived traits. The first derived trait of cercopithecoids is their bilophodont molars (“bi” meaning two, “loph” referring to ridge, and “dont” meaning tooth). If you refer back to Figure 5.33, you will see how the molars of cercopithecoids have four cusps arranged in a square pattern and have two ridges connecting them. It is thought that this molar enabled these monkeys to eat a wide range of foods, thus allowing them to live in habitats that apes cannot. The other key derived trait that all cercopithecoids share is having ischial callosities (Figure 5.34). The ischium is the part of your pelvis that you are sitting on right now (see Appendix A: Osteology). In cercopithecoids, this part of the pelvis has a flattened surface that, in living animals, has callused skin over it. These function as seat pads for cercopithecoids, who often sit above branches when feeding and resting.
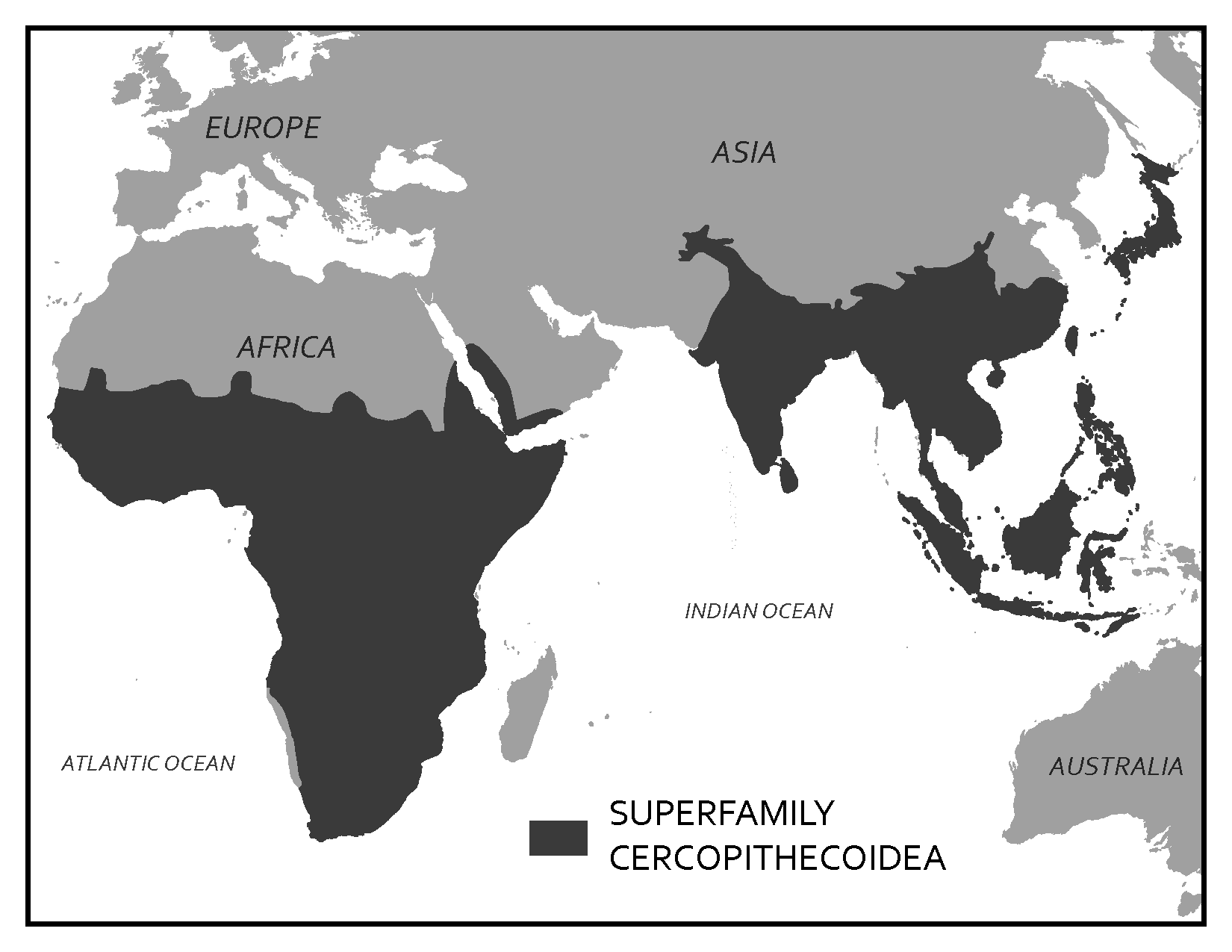
Cercopithecoid monkeys are the most geographically widespread group of nonhuman primates (Figure 5.35). Since their divergence from hominoids, this monkey group has increased in numbers and diversity due, in part, to their fast reproductive rates. On average, cercopithecoids will reproduce every one to two years, whereas hominoids will reproduce once every four to nine years, depending on the taxon.
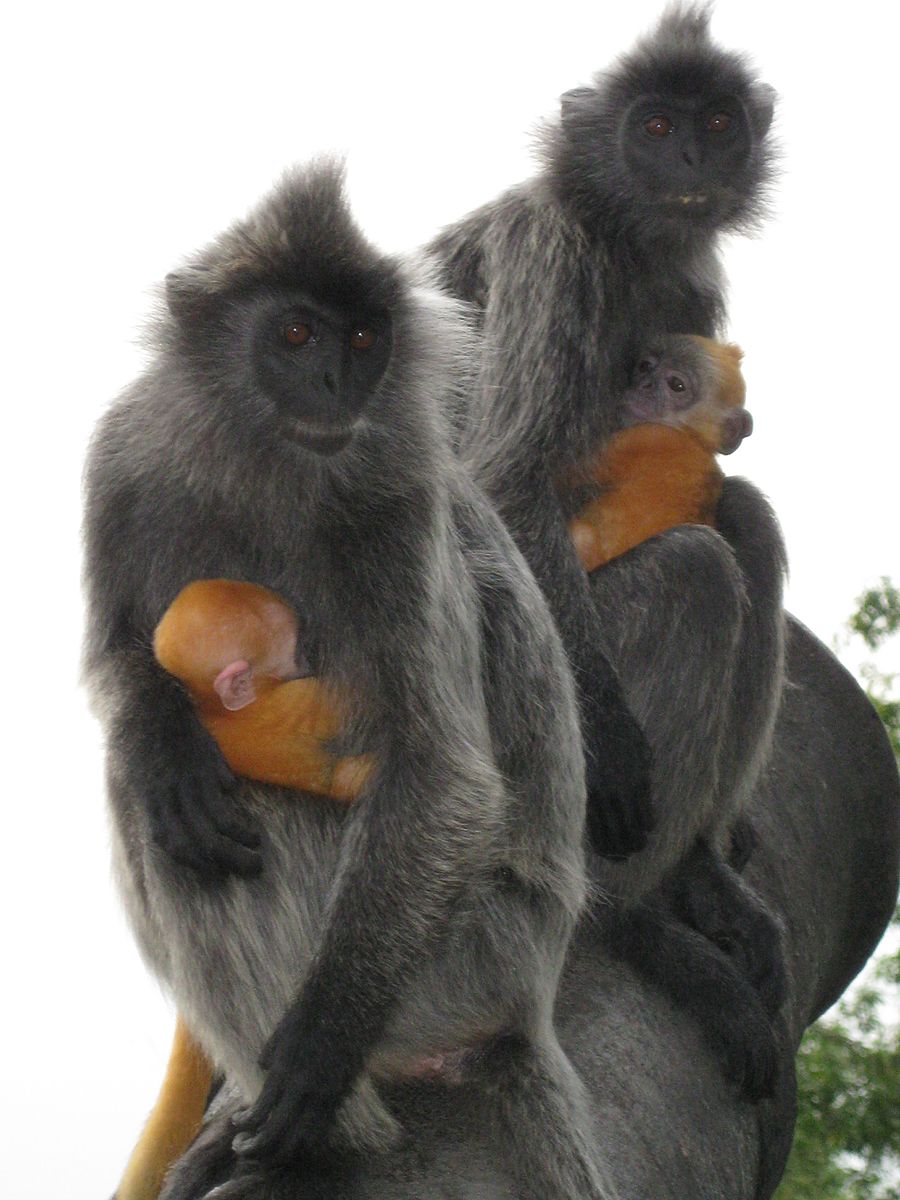
Cercopithecoidea is split into two groups, the leaf monkeys and the cheek-pouch monkeys. Both groups coexist in Asia and Africa; however, the majority of leaf monkey species live in Asia with only a few taxa in Africa. In contrast, only one genus of cheek-pouch monkey lives in Asia, and all the rest of them in Africa. As you can probably guess based on their names, the two groups differ in terms of diet. Leaf monkeys are primarily folivores, with some species eating a significant amount of seeds. Cheek-pouch monkeys tend to be more frugivorous or omnivorous, with one taxon, geladas, eating primarily grasses. The two groups also differ in some other interesting ways. Leaf monkeys tend to produce infants with natal coats—infants whose fur is a completely different color from their parents (Figure 5.36). Leaf monkeys are also known for having odd noses (Figure 5.37), and so they are sometimes called “odd-nosed monkeys.” Cheek-pouch monkeys are able to pack food into their cheek pouches (Figure 5.38), thus allowing them to move to a location safe from predators or aggressive individuals of their own species where they can eat in peace.
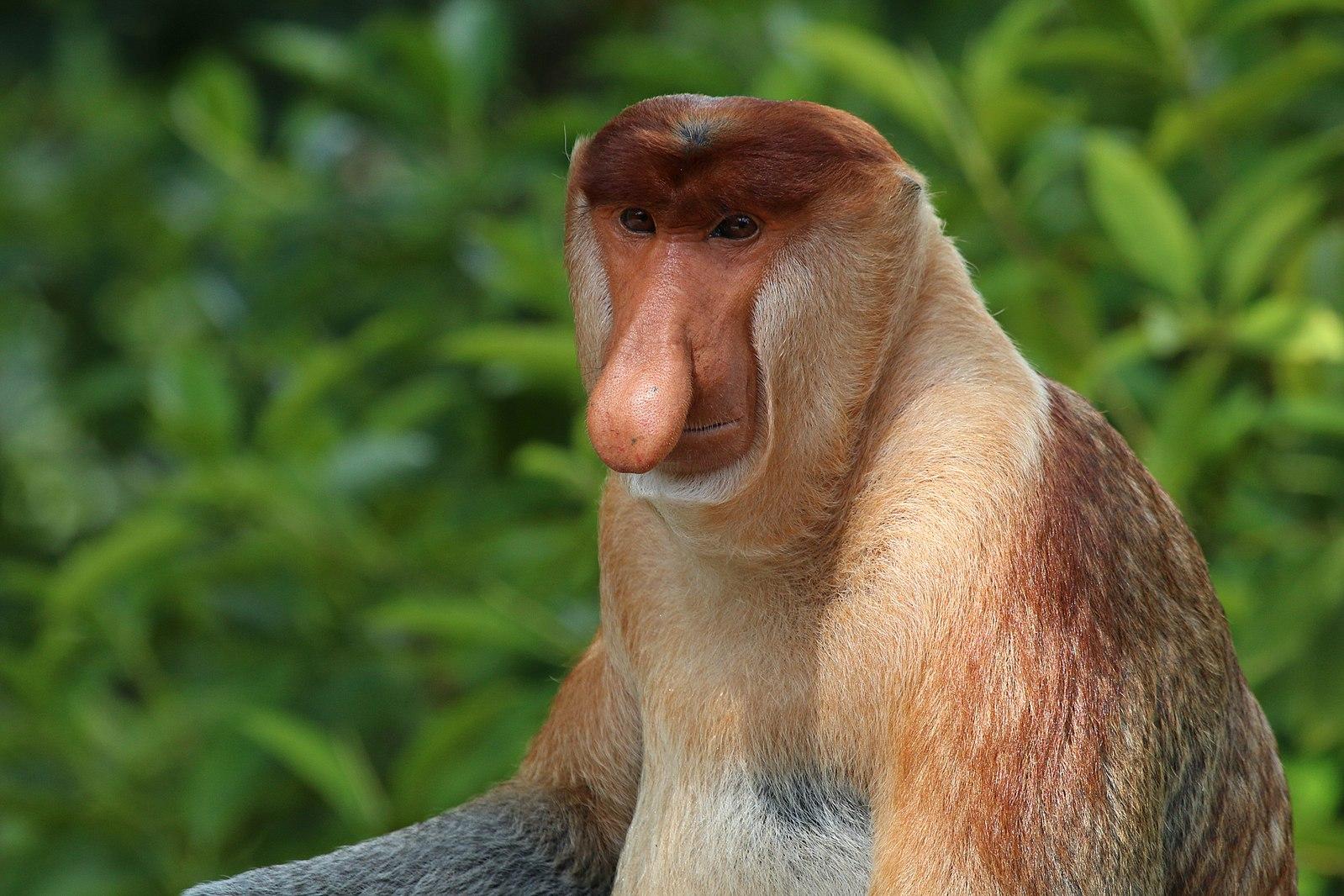
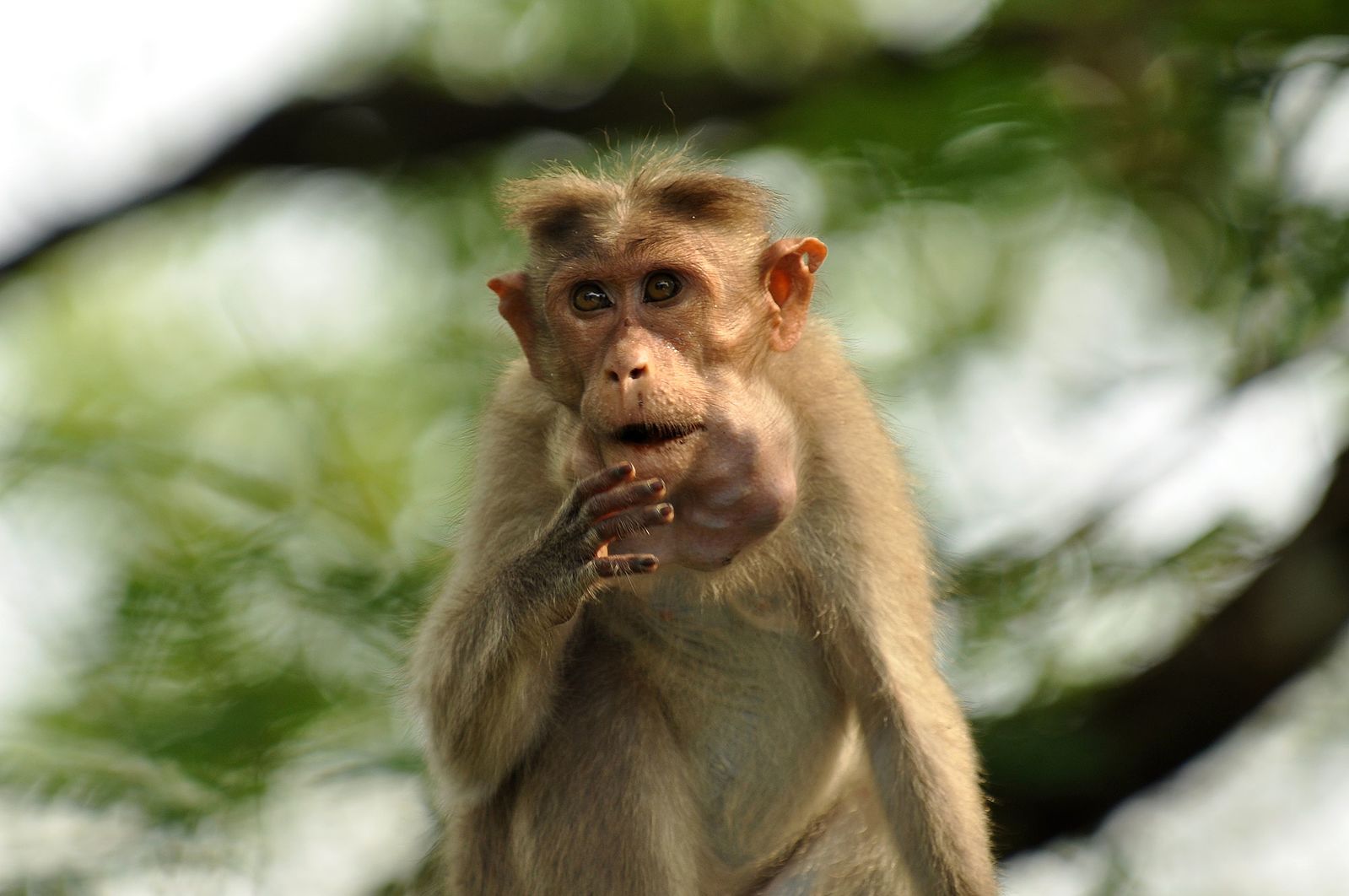
Superfamily Hominoidea of Africa and Asia
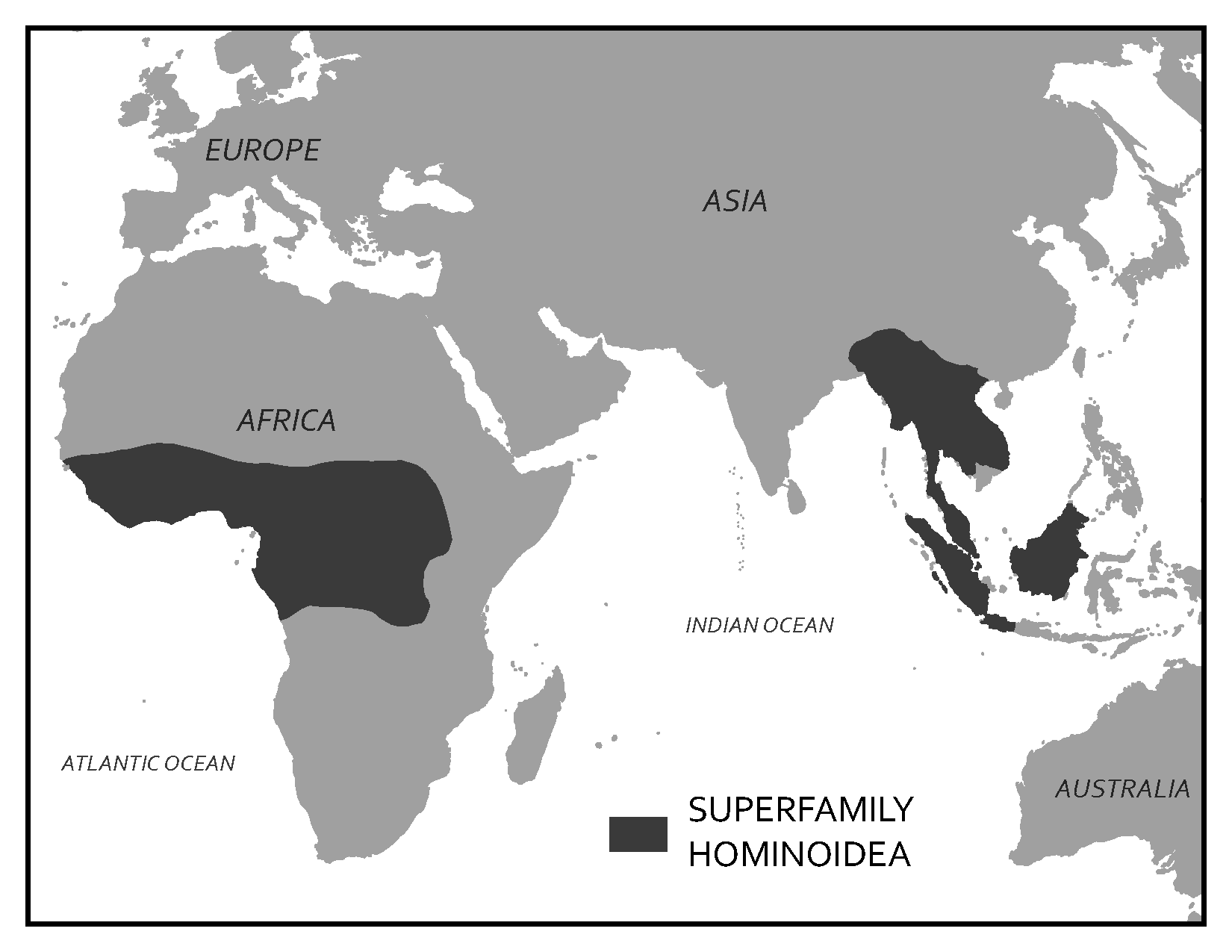
Superfamily Hominoidea of Africa and Asia (Figure 5.39) includes the largest of the living primates: apes and humans. Whereas cercopithecoid monkeys have bilophodont molars, hominoids have the more ancestral Y-5 molars, which feature five cusps separated by a “Y”-shaped groove pattern (see Figure 5.33). The Y-5 molar was present in the common ancestors of hominoids and cercopithecoids, thus it is the more ancestral molar pattern of the two. Hominoids differ the most from other primates in our body plans, due to the unique form of locomotion that hominoids are adapted for: brachiation (Figure 5.40).
To successfully swing below branches, many changes to the body needed to occur. Hominoid arms are much longer than the legs to increase reach, and the lower back is shorter and less flexible to increase control when swinging. The torso, shoulders, and arms of hominoids have evolved to increase range of motion and flexibility (see again Figure 5.12). The clavicle, or collar bone, is longer to stabilize the shoulder joint out to the side, thus enabling us to rotate our arms 360 degrees. Hominoid rib cages are wider side to side and shallower front to back than those of cercopithecoids and we do not have tails, as tails are useful for balance when running on all fours but generally not useful while swinging. Hominoids also have modified ulnae, one of the two bones in the forearm (see Appendix A: Osteology). At the elbow end of the ulna, hominoids have a short olecranon process, which allows for improved extension in our arms. At the wrist end of the ulna, hominoids have a short styloid process, which enables us to have very flexible wrists, a trait critical for swinging. Both the olecranon process and styloid process are long in quadrupedal animals who carry much of their weight on their forelimbs when traveling and who therefore need greater stability rather than flexibility in those joints.
|
Quadrupedalism |
Brachiation |
Arm length vs. leg length |
About equal |
Arms are longer |
Shoulder position |
More on the front |
Out to the side |
Ribcage shape |
Deep front-to-back Narrow side-to-side |
Shallow front-to-back Wide side-to-side |
Length of lower back |
Long |
Short |
Collar bone length |
Short |
Long |
Ulnar olecranon process |
Long |
Short |
Ulnar styloid process |
Long |
Short |
Tail |
Short to long |
None |
Apes and humans also differ from other primates in behavior and life history characteristics. Hominoids all seem to show some degree of female dispersal at sexual maturity but, as you will learn in Chapter 6, it is more common that males leave. Some apes show males dispersing in addition to females, but the hominoid tendency for female dispersal is a bit unusual among primates. Our superfamily is also characterized by the most extended life histories of all primates. All members of this group take a long time to grow and reproduce much less frequently compared to cercopithecoids. The slow pace of this life history is likely related to why hominoids have decreased in diversity since they first evolved. Figure 5.41 summarizes the key traits of Infraorder Catarrhini and its two superfamilies. Today, there are only five types of hominoids left: gibbons and siamangs, orangutans, gorillas, chimpanzees and bonobos, and humans.
Infraorder Catarrhini |
|
Downward facing, tear-drop shaped nostrils, close together Arboreal and more terrestrial taxa On average, largest primates On average, most sexually dimorphic taxonomic group 2:1:2:3 dental formula All trichromatic |
|
|
Wide geographic distribution Bilophodont molars Ischial callosities Reproduce every 1–2 years |
Tropical forests of Africa and Asia Y-5 molars Adaptations for brachiation Reproduce every 4–9 years |
Family Hylobatidae of Southeast Asia
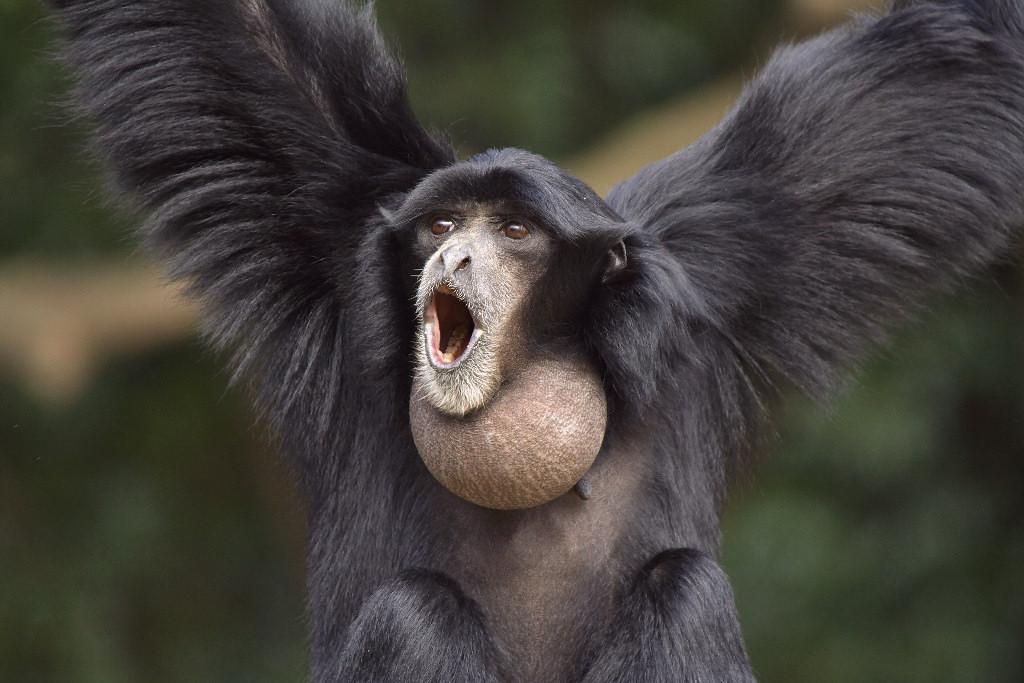
The number of genera in this group has been changing in recent years, but the taxa broadly encompasses gibbons and siamangs. Both are found across Southeast Asian tropical forests. Gibbons weigh, on average, about 13 pounds and tend to be more frugivorous, whereas siamangs are larger than gibbons and also more folivorous. Unlike the larger-bodied apes (orangutans, chimps, bonobos, and gorillas) who make nests to sleep in every night, gibbons and siamangs will develop callused patches on their ischium resembling ischial callosities. Gibbon species are quite variable in their coloration and markings, while siamangs are all black with big throat sacs that are used in their exuberant vocalizations (Figure 5.42). Both gibbons and siamangs live in pairs with very little sexual dimorphism, although males and females do differ in coloration in some gibbon species.
Pongo of Southeast Asia
The Genus Pongo refers to orangutans. These large red apes are found in Southeast Asia, with the two well-known species each living on the islands of Borneo and Sumatra. A third, very rare species, was recently discovered in Southern Sumatra (Nater et al. 2017). Orangutans are highly frugivorous but will supplement their diet with leaves and bark when fruit is less available. As mentioned earlier, orangutans are the only diurnal, solitary taxon among primates and are extremely slow to reproduce, producing only one offspring about every seven to nine years. They are highly sexually dimorphic (Figure 5.43 a and b), with fully developed, “flanged” males being approximately twice the size of females. These males have large throat sacs; long, shaggy coats; and cheek flanges. The skulls of male orangutans often feature a sagittal crest, which is believed to function as additional attachment area for chewing muscles as well as a trait used in sexual competition (Balolia, Soligo, and Wood 2017). An unusual feature of orangutan biology is male bimaturism. Male orangutans are known to delay maturation until one of the more dominant, flanged males disappears. The males that delay maturation are called “unflanged” males, and they can remain in this state for their entire life. Unflanged males resemble females in their size and appearance and will sneak copulations with females while avoiding the bigger, flanged males. Flanged and unflanged male orangutans represent alternative reproductive strategies, both of which successfully produce offspring (Utami et al. 2002).
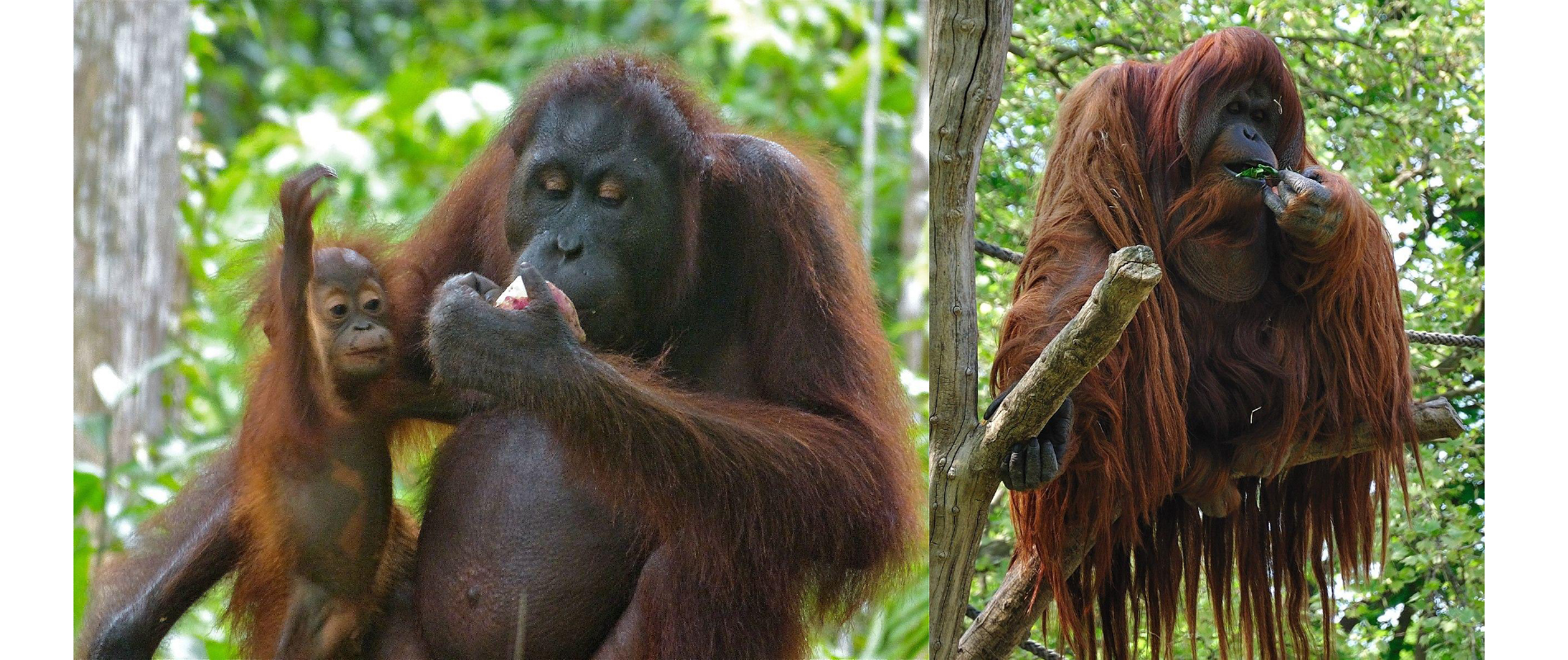
Gorilla of Africa
There are several species of gorillas that can be found across Central Africa. Gorilla males, like orangutan males, are about twice the size of female gorillas (Figure 5.44a and b). When on the ground, gorillas use a form of quadrupedalism called knuckle-walking, wherein the fingers are curled under and the weight is carried on the knuckles. Male gorillas have a large sagittal crest and large canines compared with females. Adult male gorillas are often called “silverbacks” because when they reach about twelve to thirteen years old, the hair on their backs turns silvery gray. Gorillas typically live in groups of one male and several females. Gorillas are considered folivorous, although some species can be more frugivorous depending on fruit seasonality (Remis 1997).
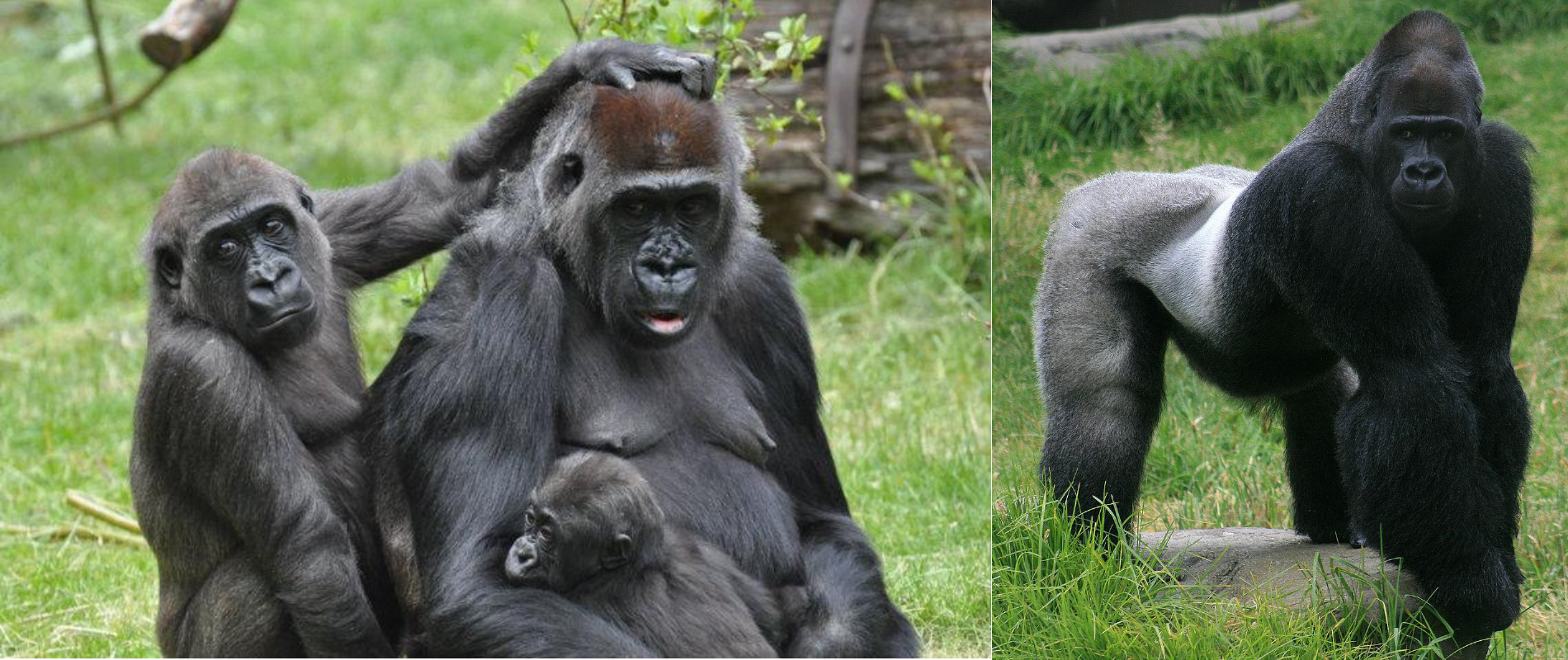
Pan of Africa
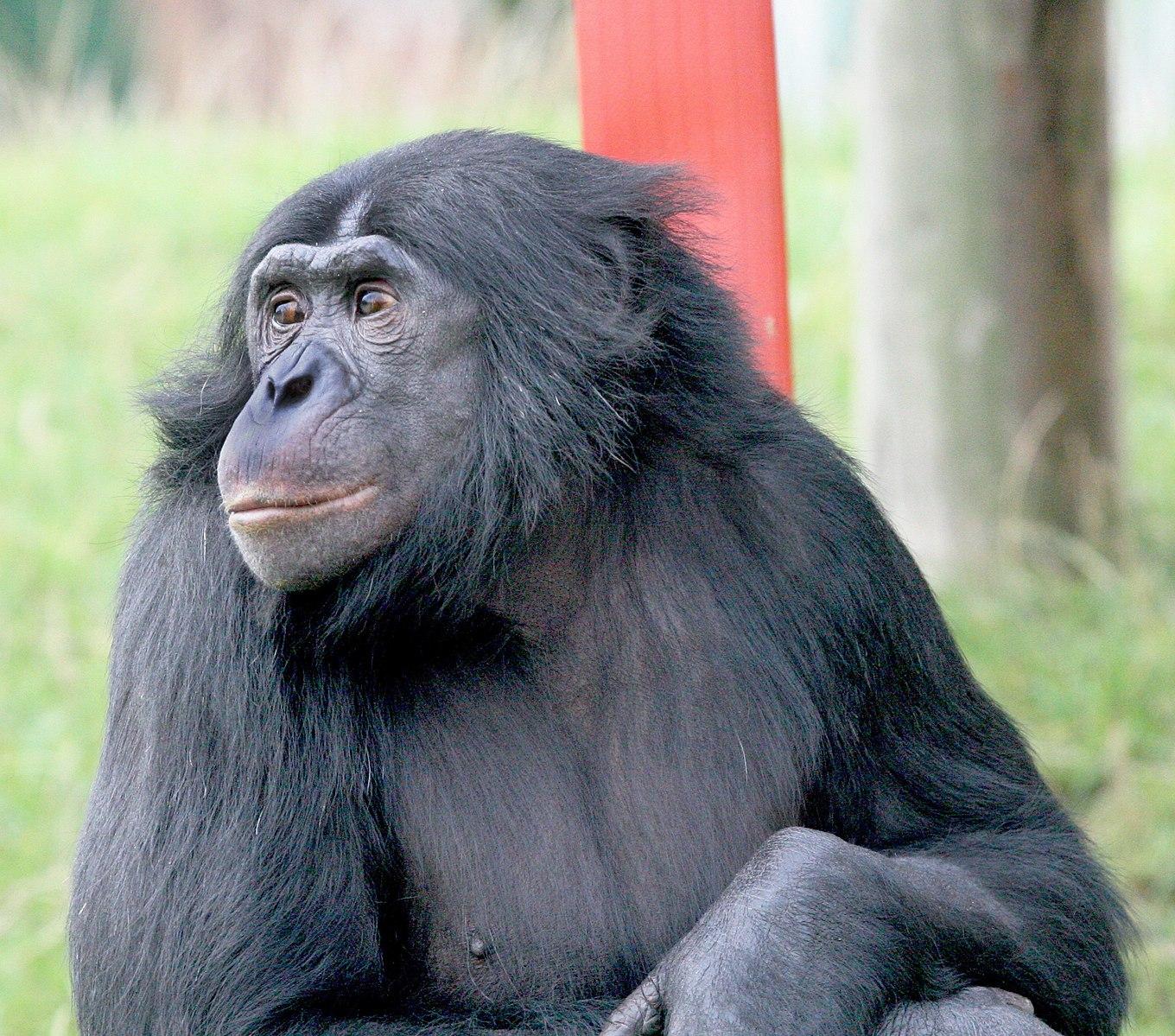
The Genus Pan includes two species: Pan troglodytes (the common chimpanzee) and Pan paniscus (the bonobo). These species are separated by the Congo River, with chimpanzees ranging across West and Central Africa and bonobos located in a restricted area south of the Congo River. Chimpanzees and bonobos both have broad, largely frugivorous diets.The two species differ morphologically in that bonobos are slightly smaller, have their hair parted down the middle of their foreheads, and are born with dark faces (Figure 5.45). In contrast, chimpanzees do not have the distinctive parted hair and are born with light faces that darken as they mature (Figure 5.46). Chimpanzees and bonobos live in a grouping called a fission-fusion community, which you will learn more about in Chapter 6. Both species are moderately sexually dimorphic, with males about 20% larger than females. When on the ground, chimpanzees and bonobos knuckle-walk like gorillas do.
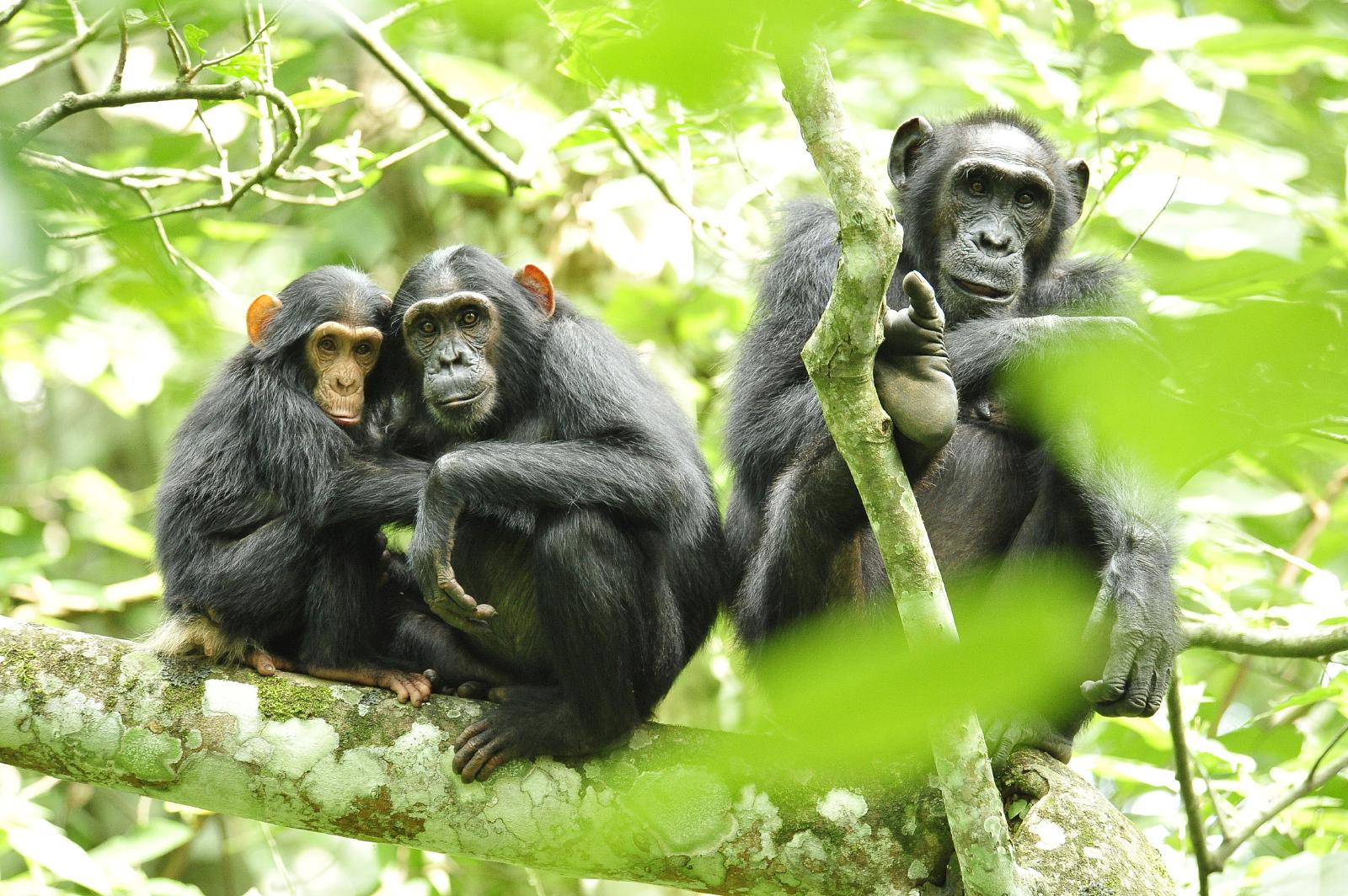
Homo
The last member of the Hominoidea to discuss is our own taxon, Genus Homo. Later chapters will discuss the many extinct species of Homo, but today there is only one living species of Homo, our own species, sapiens. While it is interesting to focus on how humans differ from apes in many aspects of our morphology, behavior, and life history, one objective of this chapter, and of biological anthropology in general, is to understand our place in nature. This means looking for aspects of human biology that link us to the taxonomic diversity we have discussed. To that end, here we will focus on similarities humans share with other hominoids.
Like other hominoids, humans lack a tail and possess upper-body adaptations for brachiation. While our lower body has been modified for a bipedal gait, we are still able to swing from branches and throw a baseball, all thanks to our mobile shoulder joint. Humans, like other hominoids, also have a Y-5 cusp pattern on our molars. All hominoids, including humans, have an extended life history, taking time to grow and develop, and reproducing slowly over a long life span. Lastly, while humans show a great deal of variation across cultures, many human societies show tendencies for female dispersal (Burton et al. 1996).
Among the hominoids, humans show particular affinities with other members of the African Clade, Pan and Gorilla. Humans share over 96% of our DNA with gorillas (Scally et al. 2012), and over 98% with Pan (Ebersberger et al. 2002). Even without this strong genetic evidence, the African Clade of hominoids share many morphological similarities, including having wide-set eye sockets and backward-sweeping cheekbones. Today, Pan and Gorilla knuckle-walk when on the ground, and it has been suggested the last common ancestor of chimpanzees, bonobos, gorillas, and humans did as well (Richmond, Begun, and Strait 2001). Further, humans, chimpanzees, and bonobos all live in fission-fusion social groups characterized by shared behaviors, like male cooperation in hunting and territoriality, as well as tool use.
Special Topic: Primates in Culture and Religion
One of the best parts of teaching anthropology for me is getting to spend time watching primates at zoos. What I also find interesting is watching people watch primates. I have very often heard a parent and child walk up to a chimpanzee enclosure and exclaim “Look at the monkeys!” The parent and child often don’t know that a chimpanzee is not a monkey, nor are they likely to know that chimpanzees share more than 98% of their DNA with us. What strikes me as significant is that, although most people do not know the difference between a monkey, an ape, and a lemur, they nonetheless recognize something in the animals as being similar to themselves. In fact, recognition of similarities between humans and other primates is very ancient, dating back far earlier than Linnaeus. For many of us, we only ever get to see primates in zoos and animal parks, but in many areas of the world, humans have coexisted with these animals for thousands of years. In areas where humans and primates have a long, shared history, nonhuman primates often play key roles in creation myths and cultural symbolism.
Hamadryas baboons feature significantly in Ancient Egyptian iconography. Ancient Egyptian deities and beliefs transformed over time, as did the role of hamadryas baboons. Early on, baboons were thought to represent dead ancestors, and one monkey deity, called Babi or Baba, was thought to feed off of dead souls. Later, baboons became the totem animal for Thoth, the deity of science, writing, wisdom, and measurement, who also wrote the Book of the Dead. Sunbathing hamadryas baboons led ancient Egyptians to associate them with Ra, the sun god, who was the son of Thoth. During mummification, human organs were removed and put into canopic jars, one of which was topped with the head of the baboon-headed god, Hapi. Hamadryas baboons were also often kept as pets, as depicted in hieroglyphics, and occasionally mummified as well.
On Madagascar, indris and aye-ayes play roles in the creation myths and omens of local people.There are many myths regarding the origins of indris and their relationship to humans, including one where two brothers living in the forest separated, with one brother leaving the forest and becoming a human while the other stayed in the forest to become the indri. Like humans, indris have long legs, no tail, and upright posture. They are considered sacred and are therefore protected. Unfortunately, the aye-aye is not treated with the same reverence. Because of their unusual appearance (see Figure 5.15), aye-ayes are seen as omens of death.They are usually killed when encountered because it is believed that someone will die if an aye-aye points at them.
In India, monkeys play a key role in the Hindu religion. Hanuman, who resembles a monkey, is a key figure in the Ramayana. Hanuman is thought to be a guardian deity, and so local monkeys like Hanuman langurs and macaques are protected in India (Figure 5.47). In Thailand, where Hinduism is also practiced, the Hindu reverence for monkeys extends to “monkey feasts,” where large quantities of food are spread out in gratitude to the monkeys for bringing good fortune.
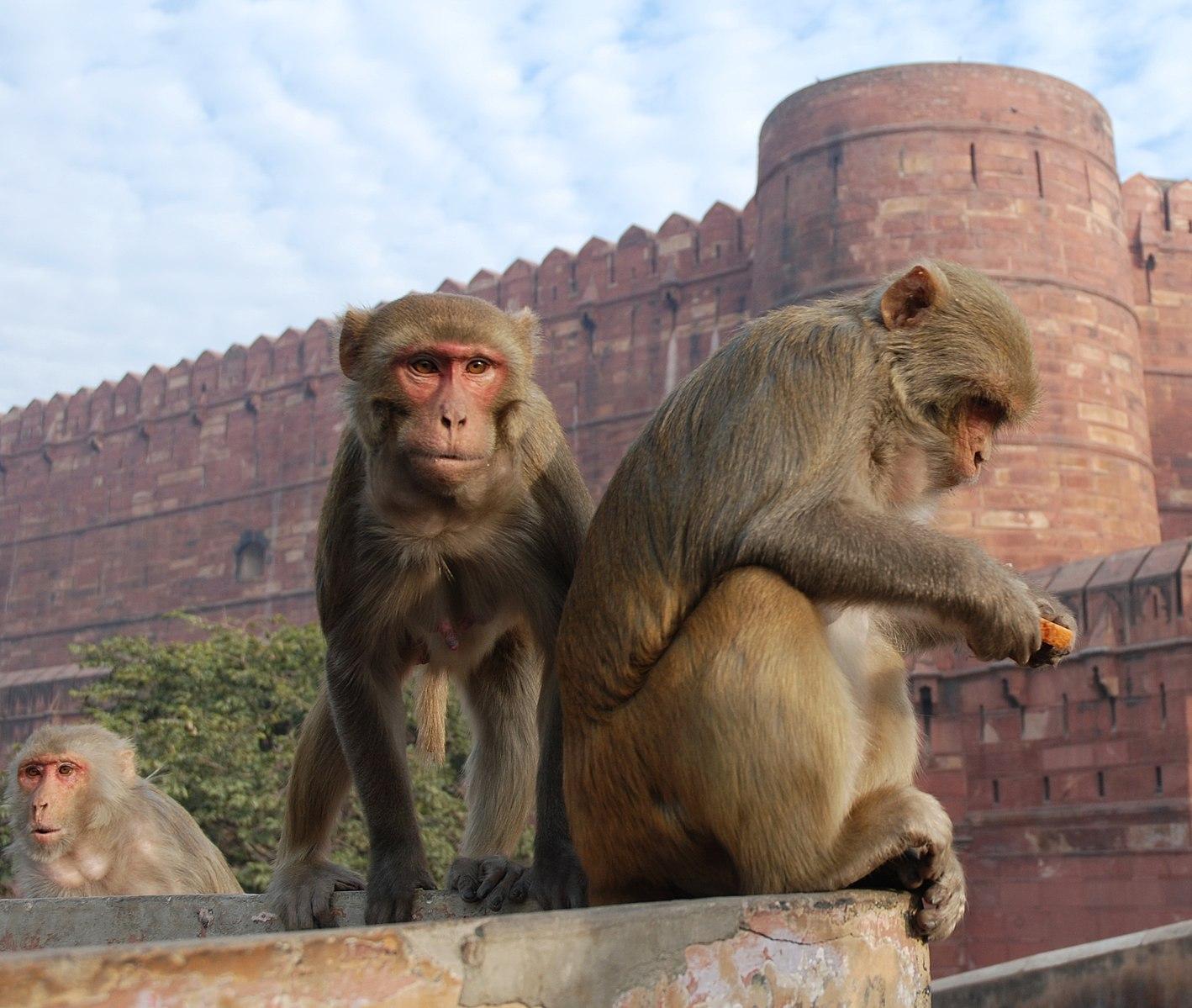
The people of Japan have coexisted with Japanese macaques for thousands of years, and so monkeys play key roles in both of the major Japanese religions. In the Shinto religion, macaques are thought of as messengers between the spirit world and humans, and monkey symbols are thought to be good luck. The other major religion in Japan is Buddhism, and monkeys play a role in symbolism of this religion as well. The “Three Wise Monkeys” who see no evil, speak no evil, and hear no evil derive from Buddhist iconography of monkeys.
In Central and South America, monkeys feature often in Mayan and Aztec stories. In the Mayan creation story, the Popol Vuh, the “hero brothers,” are actually a howler monkey and a spider monkey, who represent ancestors of humans in the story. In the Aztec religion, spider monkeys are associated with the god of arts, pleasure, and playfulness. A spider monkey is also represented in a Peruvian Nazca geoglyph, a large design made on the ground by moving rocks.
In many of these regions today, the relationships between humans and nonhuman primates are complicated. The bushmeat and pet trades make these animals valuable at the expense of many animals’ lives, and in some areas, nonhuman primates have become pests who raid crop fields and consume valuable foods. All of this has led to the development of a new subarea of anthropology called Ethnoprimatology, which involves studying the political, economic, symbolic, and practical relationships between humans and nonhuman primates.This field highlights the particular challenges for humans of having to coexist with animals with whom we share so much in common. It also provides insight into some of the challenges facing primate conservation efforts (see Appendix B: Primate Conservation).
Conclusion
The Order Primates is a diverse and fascinating group of animals united in sharing a suite of characteristics—visual specialization, grasping hands and feet, large brains, and extended life histories—that differentiates us from other groups of mammals. In this chapter, we surveyed the major taxonomic groups of primates, discussing where humans fit among our close relatives as well as discovering that primates are interesting animals in their own right. We discussed a range of key traits used to distinguish between the many taxa of living primates, including dietary, locomotor, and behavioral characteristics. Because of our long, shared evolutionary history with these animals, nonhuman primates provide a crucial resource for understanding our current biology. In Chapter 6, you will discover the fascinating and complex social behaviors of nonhuman primates, which provide further insight into our evolutionary biology.
Review Questions
- Why does the field of anthropology, a field dedicated to the study of humans, include the study of nonhuman animals? What can we learn from nonhuman primates?
- Why is it important to try to place taxa into a clade classification rather than groupings based on grade? Can you think of an example?
- One of the important goals of an introductory biological anthropology course is to teach you about your place in nature. What is the full taxonomic classification of humans, and what are some of the traits we have of each of these categories?
- When you have seen primates in person, did you observe any facial expressions, behaviors, or physical traits that seemed familiar to you? If so, which ones and why?
- Draw out a tree showing the major taxonomic group of primates described here, making sure to leave room in between each level. Underneath each taxon, list some of the key features of this group so that you can compare traits between groups.
Key Terms
Activity pattern: Refers to the time of day an animal is typically active.
African clade: A grouping that includes gorillas, chimpanzees, bonobos, humans, and their extinct relatives.
Analogy: When two or more taxa exhibit similar traits that have evolved independently, the similar traits evolve due to similar selective pressures. (Also sometimes called convergent evolution, parallel evolution, or homoplasy.)
Ancestral trait: A trait that has been inherited from a distant ancestor.
Arboreal: A descriptor for an organism that spends most of its time in trees.
Asian clade: A grouping that includes orangutans and their extinct relatives.
Bilophodont: Molar pattern of cercopithecoid monkeys in which there are usually four cusps that are arranged in a square pattern and connected by two ridges.
Bipedalism: Walking on two legs.
Brachiation: A form of locomotion in which the organism swings below branches using the forelimbs.
Bunodont: Low, rounded cusps on the cheek teeth.
Canines: In most primates, these are the longest of the teeth, often conical in shape and used as a weapon against predators or others of their species.
Cathemeral: Active throughout the 24-hour period.
Clade: A grouping based on ancestral relationships; a branch of the evolutionary tree.
Cusps: The bumps on the chewing surface of the premolars and molars, which can be quite sharp in some species.
Dental formula: The number of each type of tooth in one quadrant of the mouth, written as number of incisors: canines: premolars: molars.
Derived trait: A trait that has been recently modified, most helpful when assigning taxonomic classification.
Diastema: A space between the teeth, usually for large canines to fit when the mouth is closed.
Dichromatic: Being able to see only blues and greens.
Diurnal: Active during the day.
Dry nose: The nose and upper lip are separated and the upper lip can move independently; sometimes referred to as a “hairy” or “mobile” upper lip.
Ethnoprimatology: A subarea of anthropology that studies the complexities of human-primate relationships in the modern environment.
Evolutionary trade-off: When an organism, which is limited in the time and energy it can put into aspects of its biology and behavior, is shaped by natural selection to invest in one adaptation at the expense of another.
Faunivorous: Having a diet consisting entirely of animal matter: insects, eggs, lizards, etc.
Folivore: Having a diet consisting primarily of leaves.
Fovea: A depressed area in the retina at the back of the eye containing a concentration of cells that allow one to focus on objects very close to one’s face.
Frugivore: Having a diet consisting primarily of fruit.
Generalized trait: A trait that is useful for a wide range of tasks.
Grade: A grouping based on overall similarity in lifestyle, appearance, and behavior.
Grooming claw: A claw present on the second pedal digit in strepsirrhines.
Gummivore: Having a diet consisting primarily of gums and saps.
Heterodont: Having different types of teeth.
Homology: When two or more taxa share characteristics because they inherited them from a common ancestor.
Hone: When primates sharpen their canines by wearing them on adjacent teeth.
Incisors: The spatula-shaped teeth at the front of the mouth.
Insectivore: Having a diet consisting primarily of insects.
Ischial callosities: Modified seat bones of the pelvis that are flattened and over which calluses form; function as seat pads for sitting and resting atop branches.
Knuckle-walking: A form of quadrupedal movement used by Gorilla and Pan when on the ground, wherein the front limbs are supported on the knuckles of the hands.
Life history: Refers to an organism’s pace of growth, reproduction, lifespan, etc.
Locomotion: How an organism moves around.
Male bimaturism: Refers to the alternative reproductive strategies in orangutans in which males can delay maturation, sometimes indefinitely, until a fully mature, “flanged” male disappears.
Molars: The largest teeth at the back of the mouth; used for chewing. In primates, these teeth usually have between three and five cusps.
Monochromatic: Being able to see only in shades of light to dark, no color.
Monomorphic: When males and females of a species do not exhibit significant sexual dimorphism.
Natal coat: Refers to the contrasting fur color of baby leaf monkeys compared to adults.
Nocturnal: Active at night.
Olecranon process: Bony projection at the elbow end of the ulna.
Opposable thumb or opposable big toe: Having thumbs and toes that go in a different direction from the rest of the fingers, allows for grasping with hands and feet.
Pentadactyly: Having five digits or fingers and toes.
Polymorphic color vision: A system in which individuals of a species vary in their abilities to see color. In primates, it refers to males being dichromatic and females being either trichromatic or dichromatic.
Postorbital bar: A bony ring that surrounds the eye socket, open at the back.
Postorbital closure/plate: A bony plate that provides protection to the side and back of the eye.
Prehensile tail: A tail that is able to hold the full body weight of an organism, which often has a tactile pad on the underside of the tip for improved grip.
Premolars: Smaller than the molars, used for chewing. In primates, these teeth usually have one or two cusps.
Quadrupedalism: Moving around on all fours.
Rhinariums: Wet noses; resulting from naked skin of the nose which connects to the upper lip and smell-sensitive structures along the roof of the mouth.
Sagittal crest: A bony ridge along the top/middle of the skull, used for attachment of chewing muscles.
Scent marking: The behavior of rubbing scent glands or urine onto objects as a way of communicating with others.
Semi-brachiation: A form of locomotion in which an organism swings below branches using a combination of forelimbs and prehensile tail.
Sexually dimorphic: When a species exhibits sex differences in morphology, behavior, hormones, and/or coloration.
Shearing crests: Sharpened ridges that connect cusps on a bilophodont molar.
Specialized trait: A trait that has been modified for a specific purpose.
Styloid process of ulna: A bony projection of the ulna at the end near the wrist.
Tactile pads: Sensitive skin at the fingertips for sense of touch. Animals with a prehensile tail have a tactile pad on the underside of the tail as well.
Tapetum lucidum: Reflecting layer at the back of the eye that magnifies light.
Terrestrial: A descriptor for an organism that spends most of its time on the ground.
Tetrachromatic: Having the ability to see reds, yellows, blues, greens, and ultraviolet.
Tooth comb or dental comb: A trait of the front, lower teeth of strepsirrhines in which, typically, the four incisors and canines are long and thin and protrude outward.
Trichromatic color vision: Being able to distinguish yellows and reds in addition to blues and greens.
Vertical clinging and leaping: A locomotor pattern in which animals are oriented upright while clinging to vertical branches, push off with hind legs, and land oriented upright on another vertical branch.
Y-5 molar: Molar cusp pattern in which five molar cusps are separated by a “Y”-shaped groove pattern.
About the Author
Stephanie Etting, Ph.D.
Sacramento City College and Sonoma State University, ettings@scc.losrios.edu
Dr. Etting became hooked on biological anthropology as a freshman at UC Davis when she took the “Introduction to Biological Anthropology” course. She obtained her Ph.D. in anthropology in 2011 from UC Davis, where she studied anti-predator behavior toward snakes in rhesus macaques, squirrel monkeys, and black-and-white ruffed lemurs. While in graduate school, Dr. Etting discovered her love of teaching and, since finishing her dissertation, has taught at UC Berkeley; Sonoma State University; UC Davis; California State University, Sacramento; and Sacramento City College.In addition to her interests in primate behavior, Dr. Etting is also very interested in primate evolution and functional anatomy.
For Further Exploration
Animal Diversity Web. This website is hosted by the Zoology Department at the University of Michigan. It has photographs of skulls, teeth, hands, arms, and feet of many primate species.
eSkeletons. This website is hosted by the Department of Anthropology at University of Texas, Austin. It is an interactive website where you can compare specific bones from different species of primates.
Fleagle, John G. 2013. Primate Adaptation and Evolution. Third edition. San Diego: Academic Press.
Fuentes, Agustín, and Kimberley J. Hockings. 2010. “The Ethnoprimatological Approach in Primatology.” American Journal of Primatology 72 (10): 841–847.
Rowe, Noel. 1996. Pictorial Guide to the Living Primates. Charlestown, RI: Pogonias Press.
Whitehead, Paul F., William K. Sacco, and Susan B. Hochgraf. 2005. A Photographic Atlas for Physical Anthropology. Englewood, CO: Morton Publishing.
References
Balolia, Katharine L., Christophe Soligo, and Bernard Wood. 2017. “Sagittal Crest Formation in Great Apes and Gibbons.” Journal of Anatomy 230 (6): 820–832.
Bininda-Emonds, Olaf R., Marcel Cardillo, Kate E. Jones, Ross D. E. MacPhee, Robin M. D. Beck, Richard Grenyer, Samantha A. Price, Rutger A. Vos, John L. Gittleman, and Andy Purvis. 2007. “The Delayed Rise of Present-Day Mammals.” Nature 446 (7135): 507–512.
Burton, Michael L., Carmella C. Moore, John W. M. Whiting, A. Kimball Romney, David F. Aberle, Juan A. Barcelo, Malcolm M. Dow, et al. 1996. “Regions Based on Social Structure.” Current Anthropology 37 (1): 87–123.
Chivers, David J., and C. M. Hladik. 1980. “Morphology of the Gastrointestinal Tract in Primates: Comparisons with Other Mammals in Relation to Diet.” Journal of Morphology 166 (3): 337–386.
Clutton-Brock, T. H., and Paul H. Harvey. 1980. “Primates, Brains, and Ecology.” Journal of Zoology 190 (3): 309–323.
Dunbar, Robin I. M. 1998. “The Social Brain Hypothesis.” Evolutionary Anthropology 6 (5): 178–190.
Ebersberger, Ingo, Dirk Metzler, Carsten Schwarz, and Svante Pääbo. 2002. “Genomewide Comparison of DNA Sequences Between Humans and Chimpanzees.” American Journal of Human Genetics 70 (6): 1490–1497.
Jameson, Natalie M., Zhuo-Cheng Hou, Kirstin N. Sterner, Amy Weckle, Morris Goodman, Michael E. Steiper, and Derek E. Wildman. 2011. “Genomic Data Reject the Hypothesis of a Prosimian Primate Clade.” Journal of Human Evolution 61 (3): 295–305.
Kawamura, Shoji, Chihiro Hiramatsu, Amanda D. Melin, Colleen M. Schaffner, Filippo Aureli, and Linda M. Fedigan. 2012. “Polymorphic Color Vision in Primates: Evolutionary Considerations.” In Post-Genome Biology of Primates, edited by H. Irai, H. Imai, and Y. Go, 93–120. Tokyo: Springer.
Matsui, Atsushi, Felix Rakotondraparany, Isao Munechika, Masami Hasegawa, and Satoshi Horai. 2009. “Molecular Phylogeny and Evolution of Prosimians Based on Complete Sequences of Mitochondrial DNAs.” Gene 441 (1–2): 53–66.
Nater, Alexander, Maja P. Mattle-Greminger, Anton Nurcahyo, Matthew G. Nowak, Marc de Manuel, Tariq Desai, Colin Groves, et al. 2017. “Morphometric, Behavioral, and Genomic Evidence for a New Orangutan Species.” Current Biology 27 (22): 3487–3498.
Pozzi, Luca, Jason A. Hodgson, Andrew S. Burrell, Kirstin N. Sterner, Ryan L. Raaum, and Todd R. Disotell. 2014. “Primate Phylogenetic Relationships and Divergence Dates Inferred from Complete Mitochondrial Genomes.” Molecular Phylogenetics and Evolution 75: 165–183.
Remis, Melissa J. 1997. “Western Lowland Gorillas (Gorilla gorilla gorilla) as Seasonal Frugivores: Use of Variable Resources.” American Journal of Primatology 43 (2): 87–109.
Richmond, Brian G., David R. Begun, and David S. Strait. 2001. “Origin of Human Bipedalism: The Knuckle‐Walking Hypothesis Revisited.” American Journal of Physical Anthropology 116 (S33): 70–105.
Robson, Shannen L., Carel P. van Schaik, and Kristen Hawkes. 2006. “The Derived Features of Human Life History.” In The Evolution of Human Life History, edited by Kristen Hawkes and Richard R. Paine, 17–44. Santa Fe: SAR Press.
Scally, Aylwyn, Julien Y. Dutheil, LaDeana W. Hillier, Gregory E. Jordan, Ian Goodhead, Javier Herrero, Asger Hobolth, et al. 2012. “Insights into Hominid Evolution from the Gorilla Genome Sequence.” Nature 483 (7388): 169–175.
Schneider, Horacio, and Iracilda Sampaio. 2015. “The Systematics and Evolution of New World Primates: A Review.” Molecular Phylogenetics and Evolution 82 (B): 348–357.
Setchell, Joanna M., Phyllis C. Lee, E. Jean Wickings, and Alan F. Dixson. 2001. “Growth and Ontogeny of Sexual Size Dimorphism in the Mandrill (Mandrillus sphinx).” American Journal of Physical Anthropology 115 (4): 349–360.
Utami, Sri Suci, Benoît Goossens, Michael W. Bruford, Jan R. de Ruiter, and Jan A. R. A. M. van Hooff. 2002. “Male Bimaturism and Reproductive Success in Sumatran Orang-utans.” Behavioral Ecology 13 (5): 643–652.
Vasey, Natalie. 2006. “Impact of Seasonality and Reproduction on Social Structure, Ranging Patterns, and Fission–Fusion Social Organization in Red Ruffed Lemurs.” In Lemurs: Ecology and Adaptation, edited by Lisa Gould and Michelle L. Sauther, 275–304. New York: Springer.
Wright, Patricia C. 1999. “Lemur Traits and Madagascar Ecology: Coping with an Island Environment.” American Journal of Physical Anthropology 110 (S29): 31–72.
Acknowledgments
The author would very much like to thank the editors for the opportunity to contribute to this textbook, along with anonymous reviewers who provided useful feedback on earlier drafts of this chapter. She would particularly like to thank Karin Enstam Jaffe for her support and encouragement during the writing of this chapter and its revision. Most of all, the author would like to thank all of the Introduction to Biological Anthropology students that she has had over the years who have listened to her lecture endlessly on these animals that she finds so fascinating and who have helped her to hone her pedagogy in a field that she loves.
A similar trait found in different species because it was inherited from a common ancestor.
A similar trait found in different species that arose independently.
A grouping based on ancestral relationships; a branch of the evolutionary tree.
A grouping that includes gorillas, chimpanzees, bonobos, humans, and their extinct relatives.
A grouping that includes orangutans and their extinct relatives.
A grouping based on overall similarity in lifestyle, appearance, and behavior.
A trait that has been inherited from a distant ancestor.
A trait that has been recently modified, most helpful when assigning taxonomic classification.
A trait that is useful for a wide range of tasks.
Having thumbs and toes that go in a different direction from the rest of the fingers, allows for grasping with hands and feet.
A trait that has been modified for a specific purpose.
A bony ring that surrounds the eye socket, open at the back.
A bony plate that provides protection to the side and back of the eye.
A bony plate that provides protection to the side and back of the eye.
Being able to distinguish yellows and reds in addition to blues and greens.
Having the ability to see reds, yellows, blues, greens, and ultraviolet.
Being able to see only blues and greens.
When an organism, which is limited in the time and energy it can put into aspects of its biology and behavior, is shaped by natural selection to invest in one adaptation at the expense of another.
Refers to an organism’s pace of growth, reproduction, lifespan, etc.
A descriptor for an organism that spends most of its time in trees.
Having five digits or fingers and toes.
A descriptor for an organism that spends most of its time on the ground.
Sensitive skin at the fingertips for sense of touch. Animals with a prehensile tail have a tactile pad on the underside of the tail as well.
Having different types of teeth.
The spatula-shaped teeth at the front of the mouth.
Smaller than the molars, used for chewing. In primates, these teeth usually have one or two cusps.
The largest teeth at the back of the mouth; used for chewing. In primates, these teeth usually have between three and five cusps.
In most primates, these are the longest of the teeth, often conical in shape and used as a weapon against predators or others of their species.
When a species exhibits sex differences in morphology, behavior, hormones, and/or coloration.
When primates sharpen their canines by wearing them on adjacent teeth.
A space between the teeth, usually for large canines to fit when the mouth is closed.
The number of each type of tooth in one quadrant of the mouth, written as number of incisors: canines: premolars: molars.
The bumps on the chewing surface of the premolars and molars, which can be quite sharp in some species.
Organisms whose diet consists primarily of fruit.
Organisms whose diets consist primarily of insects.
Organisms whose diet consists primarily of leaves.
Having a diet consisting primarily of gums and saps.
Low, rounded cusps on the cheek teeth.
Sharpened ridges that connect cusps on a bilophodont molar.
Refers to the time of day an animal is typically active.
Active during the day.
Active at night.
Active throughout the 24-hour period.
How an organism moves around.
A locomotor pattern in which animals are oriented upright while clinging to vertical branches, push off with hind legs, and land oriented upright on another vertical branch.
Moving around on all fours.
A form of locomotion in which the organism swings below branches using the forelimbs.
A form of locomotion in which an organism swings below branches using a combination of forelimbs and prehensile tail.
A tail that is able to hold the full body weight of an organism, which often has a tactile pad on the underside of the tip for improved grip.
Walking on two legs.
A claw present on the second pedal digit in strepsirrhines.
A trait of the front, lower teeth of strepsirrhines in which, typically, the four incisors and canines are long and thin and protrude outward.
A trait of the front, lower teeth of strepsirrhines in which, typically, the four incisors and canines are long and thin and protrude outward.
Wet noses; resulting from naked skin of the nose which connects to the upper lip and smell-sensitive structures along the roof of the mouth.
The behavior of rubbing scent glands or urine onto objects as a way of communicating with others.
Reflecting layer at the back of the eye that magnifies light.
A depressed area in the retina at the back of the eye containing a concentration of cells that allow one to focus on objects very close to one’s face.
The nose and upper lip are separated and the upper lip can move independently; sometimes referred to as a “hairy” or “mobile” upper lip.
When males and females of a species do not exhibit significant sexual dimorphism.
Having a diet consisting entirely of animal matter: insects, eggs, lizards, etc.
A system in which individuals of a species vary in their abilities to see color. In primates, it refers to males being dichromatic and females being either trichromatic or dichromatic.
Being able to see only in shades of light to dark, no color.
Molar pattern of cercopithecoid monkeys in which there are usually four cusps that are arranged in a square pattern and connected by two ridges.
Modified seat bones of the pelvis that are flattened and over which calluses form; function as seat pads for sitting and resting atop branches.
Refers to the contrasting fur color of baby leaf monkeys compared to adults.
Molar cusp pattern in which five molar cusps are separated by a “Y”-shaped groove pattern.
Bony projection at the elbow end of the ulna.
A bony projection of the ulna at the end near the wrist.
A bony ridge along the top/middle of the skull, used for attachment of chewing muscles.
Refers to the alternative reproductive strategies in orangutans in which males can delay maturation, sometimes indefinitely, until a fully mature, “flanged” male disappears.
A form of quadrupedal movement used by Gorilla and Pan when on the ground, wherein the front limbs are supported on the knuckles of the hands.
A subarea of anthropology that studies the complexities of human-primate relationships in the modern environment.